Georgia Tech and Pacific Northwest National Laboratory Strengthen Research Ties
Oct 27, 2020 — Atlanta, GA
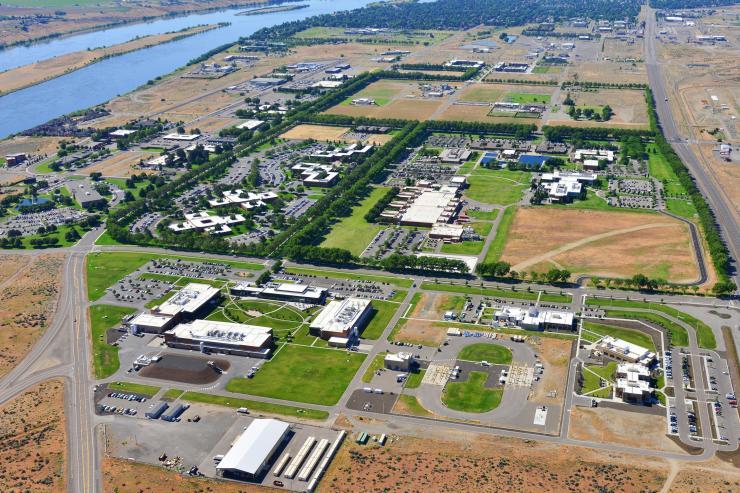
The Pacific Northwest National Laboratory's Richland Campus, seen from the north looking south. (Image courtesy of PNNL)
The Georgia Institute of Technology and the U.S. Department of Energy’s Pacific Northwest National Laboratory (PNNL) have entered into a formal agreement to bolster the interactions, collaborations, and joint scientific output of both institutions.
The goals of this collaborative arrangement are to:
- Solve Big Problems by leveraging the significant infrastructure and intellectual capabilities of both parties in a multidisciplinary and multi-institutional manner.
- Sustain and Engage Human Capital by exposing a pipeline of talented future members of the workforce to problems of practical importance and complex nature early in their academic programs.
- Accelerate Technology Adoption by introducing new ideas, science, and technology into the industrial and federal marketplace for the public good.
This five-year agreement was acknowledged during a virtual memorandum of understanding (MOU) signing event on Oct. 23, organized by Georgia Tech’s Strategic Energy Institute (SEI).
“This MOU provides a basis for both parties to engage in research collaborations, and the joint creation and administration of intellectual property,” said Tim Lieuwen, SEI’s executive director.
Leaders of both institutions emphasized that the MOU leverages existing relationships and takes advantage of synergies. PNNL and Georgia Tech already have a long history of collaboration, with more than 100 journal articles, conference papers, and the like coauthored by PNNL and Georgia Tech researchers over the past decade. PNNL also boasts 32 current staff members who earned a bachelor’s, master’s, or doctoral degree from Georgia Tech.
The MOU lays out several potential topics of mutual interest to both institutions.
“Georgia Tech and Pacific Northwest National Laboratory share interests in many areas of science and technology, including data science and visual analytics, electrical grid technologies, cybersecurity, and processing for fuels, chemicals, and materials,” said Chaouki T. Abdallah, Georgia Tech’s executive vice president for research. “Through this MOU, we look forward to expanding our collaborations in these important research areas.”
The MOU also calls for expanded intellectual engagement, with PNNL and Georgia Tech students and researchers having a substantive presence on each other’s campuses, often in the form of joint appointments and internships. Personnel exchanges of this nature typically accelerate research efforts by making available to both parties the unique capabilities, facilities, and research communities that both have to offer.
“The complexity of the research problems we are tackling today requires cooperation among institutions. No one institution can solve the big problems alone,” Tony Peurrung, PNNL’s deputy director for science and technology, said. “We are pleased to elevate our partnership with Georgia Tech because with our combined strengths, we will be better prepared to solve some of world’s most difficult science and technology challenges.”
Several online seminars are planned in the coming months to boost awareness of this agreement among the research communities of both institutions and to foster connections between researchers with similar interests.
Pacific Northwest National Laboratory draws on signature capabilities in chemistry, earth sciences, and data analytics to advance scientific discovery and create solutions to the nation's toughest challenges in energy resiliency and national security. Founded in 1965, PNNL is operated by Battelle for the U.S. Department of Energy's Office of Science — the single largest supporter of basic research in the physical sciences in the United States — and is working to address some of the most pressing challenges of our time.
The Georgia Institute of Technology, also known as Georgia Tech, is one of the nation’s leading research universities, providing a focused, technologically based education to more than 36,000 undergraduate and graduate students. The Institute has many nationally recognized programs, all top-ranked by peers and publications alike, and is ranked among the nation’s top public universities by U.S. News & World Report. It offers degrees through the Colleges of Computing, Design, Engineering, Sciences, the Scheller College of Business, and the Ivan Allen College of Liberal Arts. As a leading technological university, Georgia Tech has hundreds of centers focused on interdisciplinary research that consistently contribute vital research and innovation to American government, industry, and business.
Research News
Georgia Institute of Technology
177 North Avenue
Atlanta, Georgia 30332-0181 USA
Media Relations Contacts: Georgia Tech - John Toon (jtoon@gatech.edu); PNNL - Greg Koller (greg.koller@pnnl.gov).
John Toon
Research News
(404) 894-6986
NASA Funds Exploration into Making Rocket Fuel on Mars
Sep 18, 2020 — Atlanta, GA
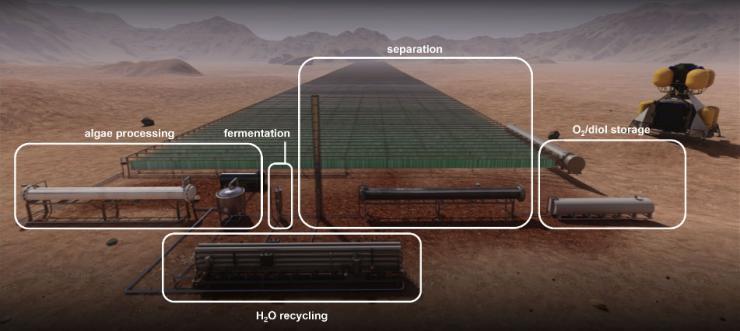
The production of a Martian biofuel from CO2 involves four modules: algae cultivation to convert CO2 to glucose, algae processing to release the stored glucose from the algal biomass, fermentation to convert glucose to the desired biofuel and separation of the biofuel for burning in a Mars ascent vehicle (MAV). Other key parts of the process include H2O recycling to maximize the use of limited Martian water and O2 storage to capture excess photosynthetic oxygen for redistribution to other parts of a Martian colony. (Picture credit: BOKO mobile study)
Nick Kruyer, a PhD candidate in the School of Chemical and Biomolecular Engineering and former RBI fellow, is part of a multi-disciplinary team exploring the renewable production of rocket fuel on Mars. The project, Fueling a Human Mission to Mars, is a collaborative effort by the groups of Caroline Genzale (Mechanical Engineering), Wenting Sun (Aerospace Engineering), and Pamela Peralta-Yahya (Chemistry and Biochemistry, PSE faculty). Dr. Genzale will present the group’s preliminary findings at next week’s NASA Innovative Advanced Concepts (NIAC) Virtual Symposium. The project is sponsored by a recently received Phase 1 NIAC funding award.
The goal of this project is to engineer production of a renewable, liquid, rocket propellant on Mars. In situ production of rocket propellant has the opportunity to reduce initial payloads from Earth, reducing launch costs by billions of dollars. The process centers around photosynthetically grown algae, cultivated using Martian carbon dioxide and sunlight, and feeding the digested algal biomass to an engineered microbe to produce rocket fuel. Preliminary research has identified diols as good fuel candidates based on their liquid state under average Martian conditions and theoretical combustion properties. The presence of oxygen atoms in the diols reduces oxidant demand and promotes a cleaner burn, potentially allowing reuse of rocket engines for multiple ascents. “It’s been really interesting to think about a chemical engineering problem in the context of Mars,” said Kruyer. “It changes all the assumptions and requires us to come up with creative solutions to problems that would already be ‘solved’ on Earth. I like the opportunity to take something science fiction and make it a reality.”
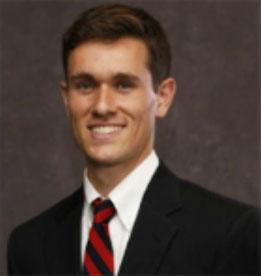
Nick Kruyer, graduate student ChBE
Walter Rich
New Process Boosts Lignin Bio-oil as a Next-Generation Fuel
Sep 14, 2020 — Atlanta, GA
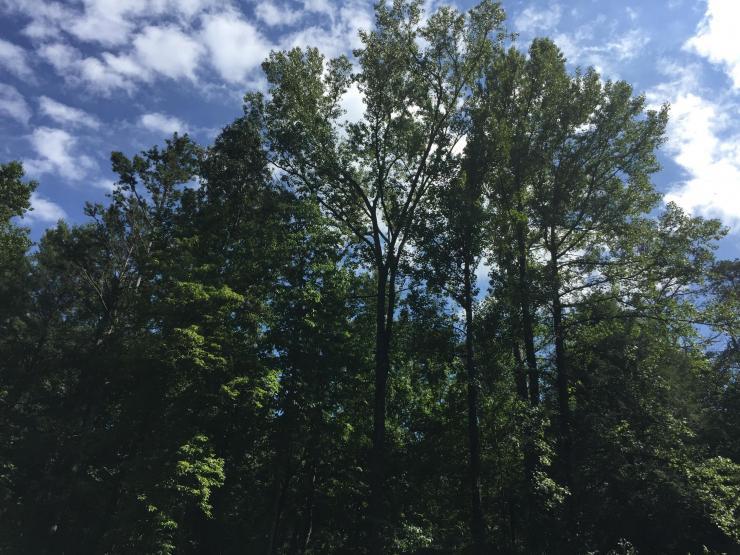
Trees are a source of cellulose, hemicelluloses, and lignin. A new process for upgrading lignin bio-oil to hydrocarbons could help expand use of the lignin, which is now largely a waste product left over from the productions of cellulose and bioethanol. (Credit: John Toon, Georgia Tech)
A new low-temperature, multi-phase process for upgrading lignin bio-oil to hydrocarbons could help expand use of the lignin, which is now largely a waste product left over from the production of cellulose and bioethanol from trees and other woody plants.
Using a dual catalyst system of superacid and platinum particles, researchers at the Georgia Institute of Technology have shown they can add hydrogen and remove oxygen from lignin bio-oil, making the oil more useful as a fuel and source of chemical feedstocks. The process, based on an unusual hydrogen cycle, can be done at low temperature and ambient pressure, improving the practicality of the upgrade and reducing the energy input needed.
“From an environmental and sustainability standpoint, people want to use oil produced from biomass,” said Yulin Deng, a professor in Georgia Tech’s School of Chemical and Biomolecular Engineering and the Renewable Bioproducts Institute. “The worldwide lignin production from paper and bioethanol manufacturing is 50 million tons annually, and more than 95% of that is simply burned to generate heat. My lab is looking for practical methods to upgrade low molecular weight lignin compounds to make them commercially viable as high-quality biofuel and biochemicals.”
The process was described September 7 in the journal Nature Energy. The research was supported by the Renewable Bioproducts Institute at Georgia Tech.
Cellulose, hemicelluloses, and lignin are extracted from trees, grasses, and other biomass materials. The cellulose is used to make paper, ethanol, and other products, but the lignin — a complex material that gives strength to the plants — is largely unused because it’s difficult to break down into low-viscosity oils that could serve as the starting point for kerosene or diesel fuel.
Pyrolysis techniques done at temperatures over 400 degrees Celsius can be used to create bio-oils such as phenols from the lignin, but the oils lack sufficient hydrogen and contain too many oxygen atoms to be useful as fuels. The current approach to addressing that challenge involves adding hydrogen and removing oxygen through a catalytic process known as hydrodeoxygenation. But that process now requires high temperatures and pressures 10 times higher than ambient, and it produces char and tar that quickly reduce the efficiency of the platinum catalyst.
Deng and colleagues set out to develop a new solution-based process that would add hydrogen and remove the oxygen from the oil monomers using a hydrogen buffer catalytic system. Because hydrogen has very limited solubility in water, the hydrogenation or hydrodeoxygenation reaction of lignin biofuel in solution is very difficult. Deng’s group used polyoxometalate acid (SiW12) as both a hydrogen transfer agent and reaction catalyst, which helps transfer hydrogen gas from the gas-liquid interphase into the bulk solution through a reversible hydrogen extraction. The process then released hydrogen as an active species H* at a platinum-on-carbon nanoparticle surface, which solved the key issue of low solubility of hydrogen in water at low pressure.
“On the platinum, the polyoxometalate acid captures the charge from the hydrogen to form H+, which is soluble in water, but the charges can be reversibly transferred back to H+ to form active H* inside the solution,” Deng said. As an apparent result, hydrogen gas is transferred to water phase to form active H*, which can directly react with lignin oil inside the solution.
In the second part of the unusual hydrogen cycle, the polyoxometalate acid sets the stage for removing oxygen from the bio-oil monomers.
“The super-acid can reduce the activation energy required for removing the oxygen, and at the same time, you have more active hydrogen H* in the solution, which reacts on the molecules of oil,” Deng said. “In the solution there is a quick reaction with active hydrogen atom H* and lignin oil on the surface of the catalyst. The reversible reaction of hydrogen with polyoxometalate to form H+ and then to hydrogen atom H* on the platinum catalyst surface is a unique reversible cycle.”
The platinum particles and polyoxometalate acid can be reused for multiple cycles without reducing efficiency. The researchers also found that the efficiency of hydrogenation and hydrodeoxygenation of lignin oil varied depending on the specific monomers in the oil.
“We tested 15 or 20 different molecules that were produced by pyrolysis and found that the conversion efficiency ranged from 50% on the lower end to 99% on the higher end,” Deng said. “We did not compare the energy input cost, but the conversion efficiency was at least 10 times better than what has been reported under similar low temperature, low hydrogen pressure conditions.”
Operating at lower temperatures — below 100 degrees Celsius — reduced the problem of char and tar formation on the platinum catalyst. Deng and his colleagues found that they could use the same platinum at least 10 times without deterioration of the catalytic activity.
Among the challenges ahead are improving the product selectivity by using different metal catalyst systems, and developing new techniques for separation and purification of the different lignin biochemicals in the solution. Platinum is expensive and in high demand for other applications, so finding a lower-cost catalyst could boost the overall practicality of the process — and perhaps make it more selective.
While helping meet the demand for bio-based oils, the new technique could also benefit the forest products, paper, and bioethanol industries by providing a potential revenue stream for lignin, which is often just burned to produce heat.
“The global lignin market size was estimated at $954.5 million in 2019, which is only a very small portion of the lignin that is produced globally. Clearly, the industry wants to find more applications for it by converting the lignin to chemicals or bio-oils,” Deng said. “There would also be an environmental benefit from using this material in better ways.”
Beyond upgrading lignin biofuel, a broad impact of the research in Yulin's group is developing a technology to significantly increase the solubility of active hydrogen atoms or hydrogen gas in a solution, which can also be used in broader chemical reactions such as ammonia synthesis and general hydrogenation of different substances.
In addition to Deng and first author Wei Liu, the research team also included Wenqin You, Wei Sun, Weisheng Yang, Akshay Korde, and Yutao Gong, all from Georgia Tech.
CITATION: Wei Liu, et al., “Ambient-pressure and low-temperature upgrading of lignin bio-oil to hydrocarbons using a hydrogen buffer catalytic system.” (Nature Energy, 2020). https://doi.org/10.1038/s41560-020-00680-x
Research News
Georgia Institute of Technology
177 North Avenue
Atlanta, Georgia 30332-0181 USA
Media Relations Contact: John Toon (404-894-6986) (jtoon@gatech.edu).
Writer: John Toon
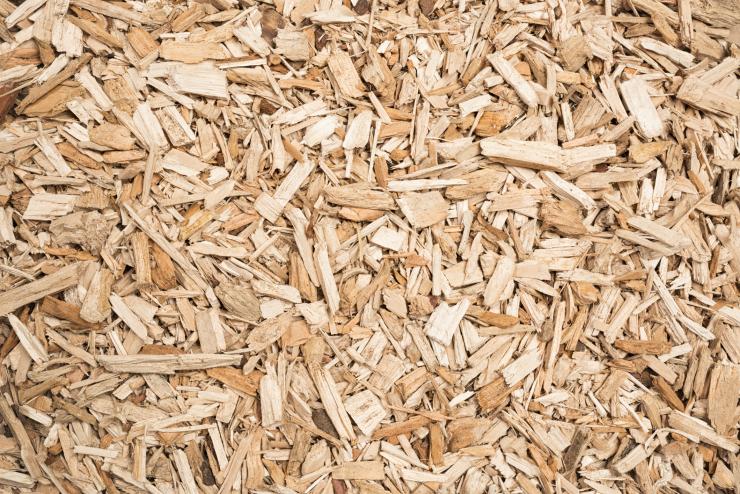
Trees are a source of cellulose, hemicelluloses, and lignin. A new process for upgrading lignin bio-oil to hydrocarbons could help expand use of the lignin, which is now largely a waste product left over from the productions of cellulose and bioethanol. (Credit: Getty Images, not for republication)
John Toon
Research News
(404) 894-6986
Flies and Mosquitoes Beware, Here Comes the Slingshot Spider
Aug 18, 2020 — Atlanta, GA
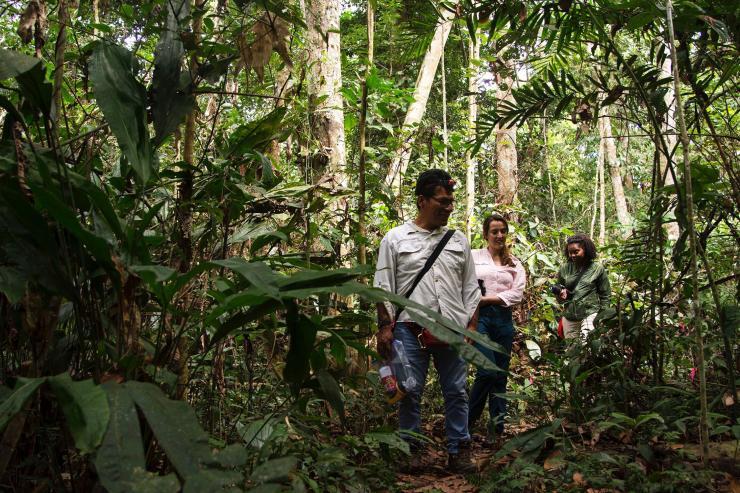
Georgia Tech researchers studied slingshot spiders in the Amazon rain forest of Peru. Shown are Jaime Navarro, a Peruvian field guide; Johanna Johnson, a Peruvian student and project volunteer; and Symone Alexander, a Georgia Tech postdoctoral researcher. (Credit: Geoff Gallice)
Running into an unseen spiderweb in the woods can be scary enough, but what if you had to worry about a spiderweb – and the spider – being catapulted at you? That’s what happens to insects in the Amazon rain forests of Peru, where a tiny slingshot spider launches a web – and itself – to catch unsuspecting flies and mosquitoes.
Researchers at the Georgia Institute of Technology have produced what may be the first kinematic study of how this amazing arachnid stores enough energy to produce acceleration of 1,300 meters/second2 – 100 times the acceleration of a cheetah. That acceleration produces velocities of 4 meters per second and subjects the spider to forces of approximately 130 Gs, more than 10 times what fighter pilots can withstand without blacking out.
The Peruvian spider and its cousins stand out among arachnids for their ability to make external tools – in this case, their webs – and use them as springs to create ultrafast motion. Their ability to hold a ready-to-launch spring for hours while waiting for an approaching mosquito suggests yet another amazing tool: a latch mechanism to release the spring.
“Unlike frogs, crickets, or grasshoppers, the slingshot spider is not relying on its muscles to jump really quickly,” said Saad Bhamla, an assistant professor in Georgia Tech’s School of Chemical and Biomolecular Engineering who studies ultrafast organisms. “When it weaves a new web every night, the spider creates a complex, three-dimensional spring. If you compare this natural silk spring to carbon nanotubes or other human-made materials in terms of power density or energy density, it is orders of magnitude more powerful.”
The study, supported by the National Science Foundation and National Geographic Society Foundation, was published August 17 in the journal Current Biology.
Understanding how web silk stores energy could potentially provide new sources of power for tiny robots and other devices, and lead to new applications for the robust material, the researchers say.
Slingshot spiders, known by the scientific genus name Theridiosomatid, build three-dimensional conical webs with a tension line attached to the center. The Peruvian member of that spider family, which is about 1 millimeter in length, pulls the tension line with its front legs to stretch the structure while holding on to the web with its rear legs. When it senses a meal within range, the spider launches the web and itself toward a fly or mosquito.
If the launch is successful, the spider quickly wraps its meal in silk. If the spider misses, it simply pulls the tension line to reset the web for the next opportunity.
“We think this approach probably gives the spider the advantage of speed and surprise, and perhaps even the effect of stunning the prey,” noted Symone Alexander, a postdoctoral researcher in Bhamla’s lab. “The spiders are tiny, and they are going after fast-flying insects that are larger than they are. To catch one, you must be much, much faster than they are.”
Slingshot spiders were described in a 1932 publication, and more recently by Jonathan Coddington, now a senior research entomologist at the Smithsonian Institution. Bhamla has an interest in fast-moving but small organisms, so he and Alexander arranged a trip to study the catapulting creature using ultrafast cameras to measure and record the movement.
“We wanted to understand these ultrafast movements because they can force our perspective to change from thinking about cheetahs and falcons as the only fast animals,” Bhamla said. “There are many very small invertebrates that can achieve fast movement through unusual structures. We really wanted to understand how these spiders achieve that amazing acceleration.”
The researchers traveled six hours by boat from Puerto Maldonado to the Tambopata Research Center. There is no electricity in the area, so nights are very dark. “We looked up and saw a tiny red dot,” Bhamla recalled. “We were so far away from the nearest light that the dot turned out to be the planet Mars. We could also see the Milky Way so clearly.”
The intense darkness raises the question of how the spider senses its prey and determines where to aim itself. Bhamla believes it must be using an acoustic sensing technique, a theory supported by the way the researchers tricked the spider into launching its web: They simply snapped their fingers.
Beyond sensing in the dark, the researchers also wondered how the spider triggers release of the web. “If an insect gets within range, the spider releases a small bundle of silk that it has created by crawling along the tension line,” Alexander said. “Releasing the bundle controls how far the web flies. Both the spider and web are moving backward.”
Another mystery is how the spider patiently holds the web while waiting for food to fly by. Alexander and Bhamla estimated that stretching the web requires at least 200 dynes, a tremendous amount of energy for a tiny spider to generate. Holding that for hours could waste a lot of energy.
“Generating 200 dynes would produce tremendous forces on the tiny legs of the spider,” Bhamla said. “If the reward is a mosquito at the end of three hours, is that worth it? We think the spider must be using some kind of trick to lock its muscles like a latch so it doesn’t need to consume energy while waiting for hours.”
Beyond curiosity, why travel to Peru to study the creature? “The slingshot spider offers an example of active hunting instead of the passive, wait for an insect to collide into the web strategy, revealing a further new functionality of spider silk,” Bhamla said. “Before this, we hadn’t thought about using silk as a really powerful spring.”
Another unintended benefit is changing attitudes toward spiders. Prior to the study, Alexander admits she had a fear of spiders. Being surrounded by slingshot spiders in the Peruvian jungle – and seeing the amazing things they do – changed that.
“In the rainforest at night, if you shine your flashlight, you quickly see that you are completely surrounded by spiders,” she said. “In my house, we don’t kill spiders anymore. If they happen to be scary and in in the wrong place, we safely move them to another location.”
Alexander and Bhamla had hoped to return to Peru this summer, but those plans were cut short by the coronavirus. They’re eager to continue learning from the spider.
“Nature does a lot of things better than humans can do, and nature has been doing them for much longer,” she said. “Being out in the field gives you a different perspective, not only about what nature is doing, but also why that is necessary.”
This research was supported by the National Science Foundation (NSF) through award 1817334 and CAREER 1941933, by the National Geographic Foundation through NGS-57996R-19, and by the Eckert Postdoctoral Research Fellowship from the Georgia Tech School of Chemical and Biomolecular Engineering. Any opinions, findings, and conclusions or recommendations expressed in this material are those of the authors and do not necessarily reflect the views of the funding organizations.
CITATION: Symone L.M. Alexander and M. Saad Bhamla, “Ultrafast launch of slingshot spiders using conical silk webs” (Current Biology, 2020). https://doi.org/10.1016/j.cub.2020.06.076
Research News
Georgia Institute of Technology
177 North Avenue
Atlanta, Georgia 30332-0181 USA
Media Relations Contact: John Toon (404-894-6986) (jtoon@gatech.edu)
Writer: John Toon
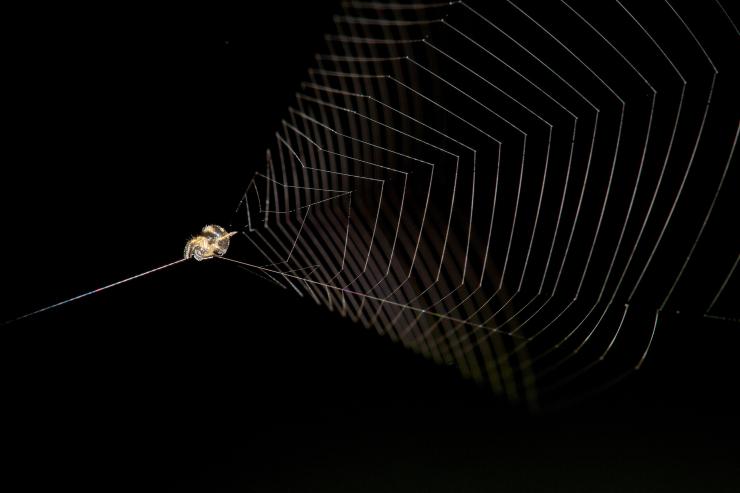
A slingshot spider is ready to launch its cone-shaped web at a flying insect. To do so, the spider will release a bundle of silk, allowing the tension line to release and catapult both the spider and the web. (Credit: Lawrence E. Reeves)
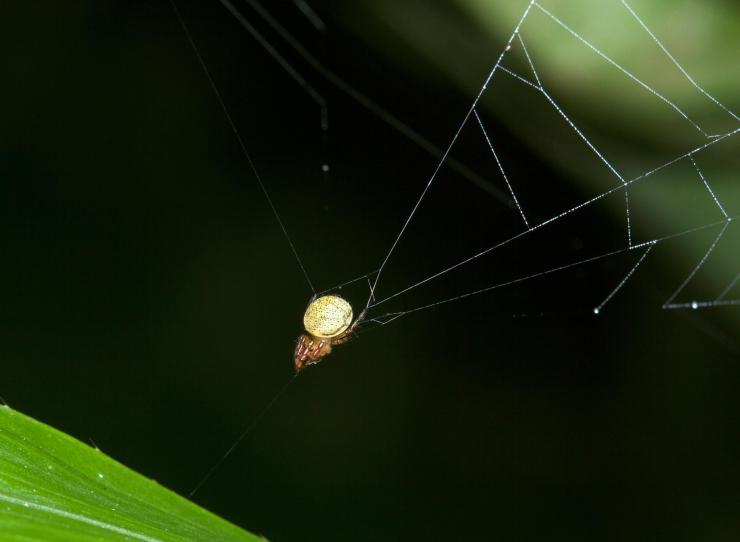
A slingshot spider is ready to launch its cone-shaped web at a flying insect. To do so, the spider will release a bundle of silk, allowing the tension line to release and catapult both the spider and the web. (Credit: Lawrence E. Reeves)
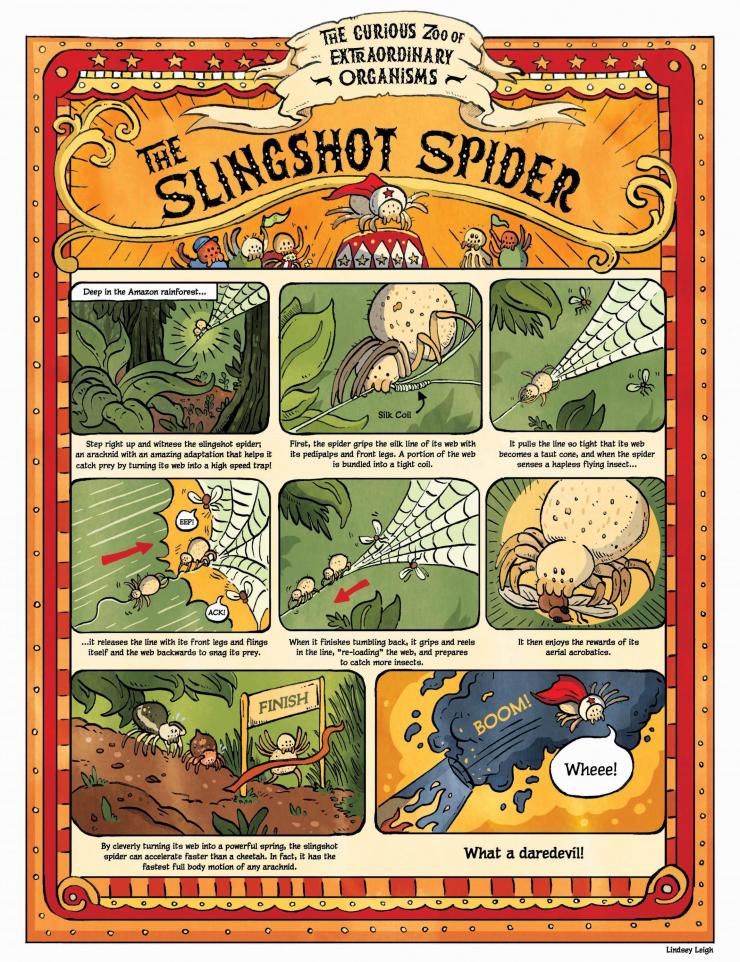
Designed for schoolchildren, this cartoon shows components of the slingshot spider's unique way of capturing insects. (Credit Lindsey Leigh)
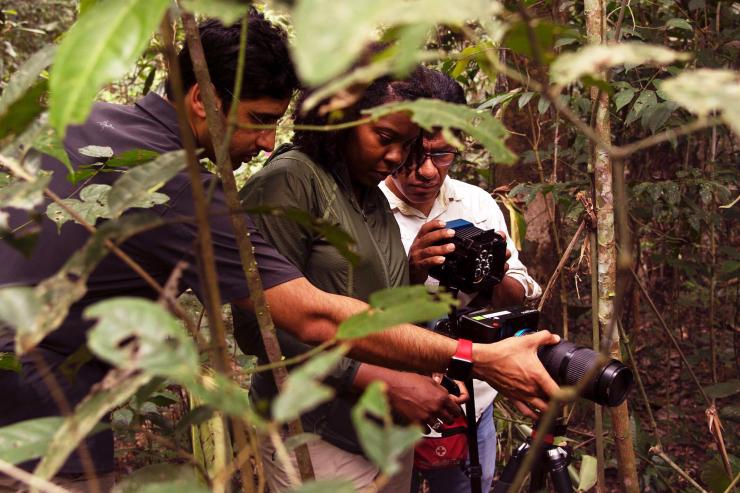
Researchers adjust an ultrafast camera in preparation for studying slingshot spiders. Shown are Assistant Professor Saad Bhamla, postdoctoral researcher Symone Alexander, and Jaime Navarro, a Peruvian field guide. (Credit: Geoff Gallice)
John Toon
Research News
(404) 894-6986
1.8 Million Face Shields Delivered to Protect Medical Workers from Covid-19
Jul 28, 2020 — Atlanta, GA
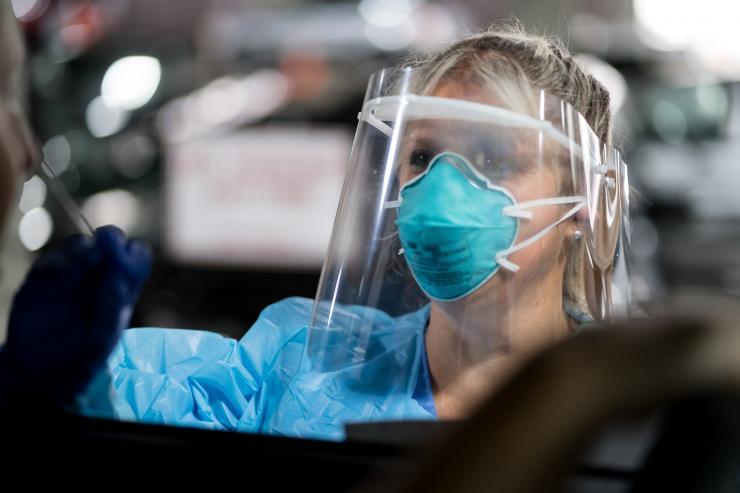
Robin Mauldin, RN, BSN, wears a face shield at Prisma Health Medical Group in Columbia, South Carolina. The shield was produced through a manufacturing effort launched by Georgia Tech and supported by a group of industrial collaborators. (Prisma Health Midlands Foundation)
Personal initiatives by a pediatrician and by researchers to make face shields for medical workers have transformed into an industry collaboration that by June had delivered 1.8 million shields to hospitals and other organizations around the country with plans to produce 2.5 million all total. A $2 million donation from Aflac Incorporated for personal protective equipment (PPE) financed the bulk of the shields.
To make it happen, a team of researchers and industry partners convened at the Global Center for Medical Innovation (GCMI), a Georgia Tech-affiliated nonprofit that guides new experimental medical solutions to market. The group combined the physician’s vision with the researchers’ original designs, adjusted them to pass FDA emergency guidelines, and then coordinated mass production and distribution.
A physician’s wisdom
The project grew wings in mid-March, after Dr. Joanna Newton became concerned that the nationwide shortage of PPE was leaving healthcare workers across the country vulnerable. Newton is a physician specializing in improving healthcare safety through technology at Children’s Healthcare of Atlanta, and she was already collaborating with Georgia Tech on other projects.
She grabbed the phone to leverage the connection.
“I called Sherry Farrugia to tell her about my idea to 3D-print PPE. We needed to quickly find a solution for the PPE shortage around the country, and I knew we had the right team here in Atlanta to help,” said Newton, a pediatric hematologist/oncologist at the Aflac Cancer and Blood Disorders Center of Children’s.
“The situation was urgent, and I knew who would have the right expertise to get this done,” said Farrugia, chief operating officer and strategy officer of Children’s Healthcare of Atlanta Pediatric Technology Center, which is part of Georgia Tech.
Farrugia had Newton present her idea at GCMI to researchers, advisors, and industry partners who immediately put together a team to address the need for face shields to protect healthcare workers from droplets containing the coronavirus. She also discussed the need with Devesh Ranjan, associate chair of the George W. Woodruff School of Mechanical Engineering, who suggested connecting the effort to a parallel initiative in that school.
Bringing in engineers
At the same time, along with Ranjan, Sam Graham, chair of the George W. Woodruff School of Mechanical Engineering, and Susan Margulies, chair of the Wallace H. Coulter Department of Biomedical Engineering, were coordinating efforts across campus to develop various medical devices in response to the pandemic. Graham, Margulies, and Ranjan quickly connected GCMI with Christopher Saldana and Saad Bhamla, faculty members in Georgia Tech’s College of Engineering, who were leading an simultaneous effort to address the face shield problem with their students using rapid fabrication techniques like 3-D printing, laser cutting, and waterjet cutting.
“The Georgia Tech mechanical engineering team used rapid fabrication equipment and quickly produced multiple face shield designs that could be manufactured in high volumes for the rapid response environment that Covid-19 required,” Saldana said.
Making a few thousand shields in a lab had likely already saved lives, but the Georgia Tech researchers and GCMI put their designs on the internet, where they have been downloaded thousands of times by organizations manufacturing them around the world. And the manufacturing partners they engaged have been turning out hundreds of thousands of shields to save many more lives.
“You may need 45 minutes for a headband with a 3D printer, but manufacturers turn out six of them every 19 seconds. Then making a million face shields becomes a real possibility,” said Mike Fisher, who leads product development at GCMI.
GCMI opened a GoFundMe page, which brought in $20,000, and then engaged their first manufacturing partner, Delta Air Lines.
A manufacturing explosion
“Delta converted one of their groups from manufacturing airplane interiors to doing the face shields. They started off by manufacturing 6,000 shields, and that got the momentum going,” Leiter said. “Two thousand shields went to Mount Sinai Hospital in New York; 2,000 went to Piedmont Healthcare in Atlanta; and 2,000 went to Children’s Healthcare of Atlanta.”
Things began to snowball.
Graham engaged Siemens Industries to fulfill a face shield order from the Georgia Emergency Management Agency (GEMA) for distribution in Georgia. Partners from ExxonMobil began looking for more potential manufacturers. And Aflac contacted Children’s looking for worthy Covid-19 related efforts to support.
“We asked for a donation of $500,000 for manufacturers to retool their operations. Aflac made a gift of $2 million to GCMI to promote the production of PPE,” Farrugia said. “We were able to buy tooling for an automotive plastics manufacturer called Quality Model in South Carolina, and they have made over 750,000 face shields so far.”
GCMI won a bid from the Federal Emergency Management Agency (FEMA) for 1,141,600 face shields, which are being made by Quality Model, where ExxonMobil helped rearrange production lines for shields.
Siemens made an additional 100,000 shields from Aflac’s gift, which is also being used to purchase existing PPE to donate to healthcare workers. Kia Motors quickly produced an initial 15,000 shields, which the company financed itself.
“Kia got the open source design from the Georgia Tech website and ran with it on their own,” Saldana said.
These partners are delivering the following number of shields: Quality Model, 1,251,600; Kia Motors, 300,000; Siemens Industries, 205,000; Delta Air Lines, 106,100; Georgia Tech, 20,000; and EIS, 15,000. And more are still to come.
The shields went across the country, from hospitals in New York City to Prisma Health in South Carolina, to nursing homes in the Pensacola area, and to rural Louisiana and Mississippi, Leiter said.
Thanks in large part to Aflac’s gift, GCMI and Farrugia are coordinating with partners, including Georgia Tech engineers, to produce N95 masks, hospital gowns, and hand sanitizer, all redesigned for the Covid-19 age.
Research News
Georgia Institute of Technology
177 North Avenue
Atlanta, Georgia 30332-0181 USA
Media Relations Assistance: John Toon (404-894-6986) (jtoon@gatech.edu).
Writer: Ben Brumfield
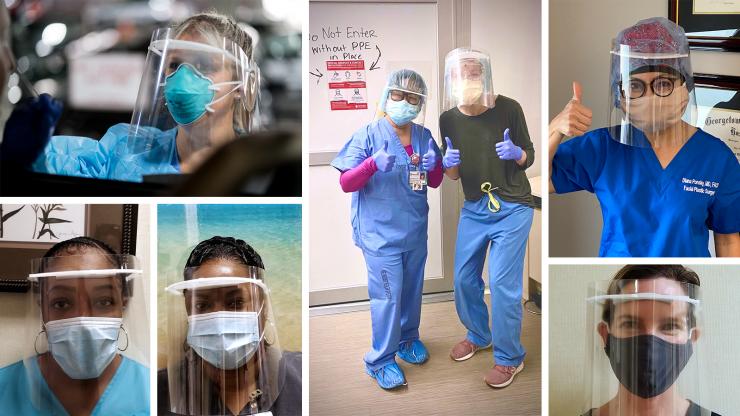
Composite image shows healthcare workers using face shields produced in a major manufacturing effort organized by Georgia Tech and the Global Center for Medical Innovation.The effort was supported by a group of industry collaborators. (Composite image: Joshua Stewart)
John Toon
Research News
(404) 894-6986
Membrane Technology Could Cut Emissions and Energy Use in Oil Refining
Jul 16, 2020 — Atlanta, GA
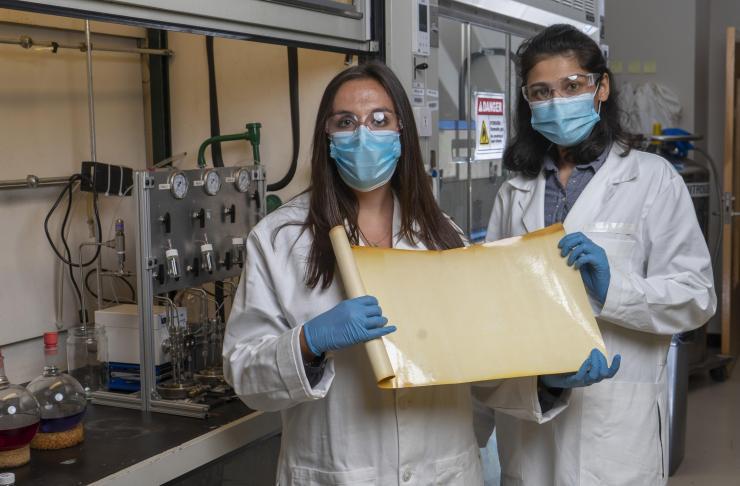
Georgia Tech graduate research assistants Kirstie Thompson and Ronita Mathias with a sample of a new membrane material that could reduce carbon emissions and energy intensity associated with refining crude oil. (Credit: Christopher Moore, Georgia Tech)
New membrane technology developed by a team of researchers from the Georgia Institute of Technology, Imperial College London, and ExxonMobil could help reduce carbon emissions and energy intensity associated with refining crude oil. Laboratory testing suggests that this polymer membrane technology could replace some conventional heat-based distillation processes in the future.
Fractionation of crude oil mixtures using heat-based distillation is a large-scale, energy-intensive process that accounts for nearly 1% of the world’s energy use: 1,100 terawatt-hours per year (TWh/yr), which is equivalent to the total energy consumed by the state of New York in a year. By substituting the low-energy membranes for certain steps in the distillation process, the new technology might one day allow implementation of a hybrid refining system that could help reduce carbon emissions and energy consumption significantly compared to traditional refining processes.
“Much in our modern lives comes from oil, so the separation of these molecules makes our modern civilization possible,” said M.G. Finn, professor and chair of Georgia Tech’s School of Chemistry and Biochemistry. Finn also holds the James A. Carlos Family Chair for Pediatric Technology. “The scale of the separation required to provide the products we use is incredibly large. This membrane technology could make a significant impact on global energy consumption and the resulting emissions of petroleum processing.”
Reported in the July 17 issue of the journal Science, the paper is believed to be the first report of a synthetic membrane specifically designed for the separation of crude oil and crude-oil fractions. Additional research and development will be needed to advance this technology to industrial scale.
Membrane technology is already widely used in such applications as seawater desalination, but the complexity of petroleum refining has until now limited the use of membranes. To overcome that challenge, the research team developed a novel spirocyclic polymer that was applied to a robust substrate to create membranes able to separate complex hydrocarbon mixtures through the application of pressure rather than heat.
Membranes separate molecules from mixtures according to differences such as size and shape. When molecules are very close in size, that separation becomes more challenging. Using a well-known process for making bonds between nitrogen and carbon atoms, the polymers were constructed by connecting building blocks having a kinked structure to create disordered materials with built-in void spaces.
The team was able to balance a variety of factors to create the right combination of solubility – to enable membranes to be formed by simple and scalable processing – and structural rigidity – to allow some small molecules to pass through more easily than others. Unexpectedly, the researchers found that the materials needed a small amount of structural flexibility to improve size discrimination, as well as the ability to be slightly “sticky” toward certain types of molecules that are found abundantly in crude oil.
After designing the novel polymers and achieving some success with a synthetic gasoline, jet fuel, and diesel fuel mixture, the team decided to try to separate a crude oil sample and discovered that the new membrane was quite effective at recovering gasoline and jet fuel from the complex mixture.
“We were initially trying to fractionate a mixture of molecules that were too similar,” said Ben McCool, a senior research associate at ExxonMobil and one of the paper’s coauthors. “When we took on a more complex feed, crude oil, we got fractionalization that looked like it could have come from a distillation column, indicating the concept’s great potential.”
The researchers worked collaboratively, with polymers designed and tested at Georgia Tech, then converted to 200-nanometer-thick films, and incorporated into membrane modules at Imperial using a roll-to-roll process. Samples were then tested at all three organizations, providing multi-lab confirmation of the membrane capabilities.
“We have the foundational experience of bringing organic solvent nanofiltration, a membrane technology becoming widely used in pharmaceuticals and chemicals industries, to market,” said Andrew Livingston, professor of chemical engineering at Imperial. “We worked extensively with ExxonMobil and Georgia Tech to demonstrate the scalability potential of this technology to the levels required by the petroleum industry.”
The research team created an innovation pipeline that extends from basic research all the way to technology that can be tested in real-world conditions.
“We brought together basic science and chemistry, applied membrane fabrication fundamentals, and engineering analysis of how membranes work,” said Ryan Lively, associate professor and John H. Woody faculty fellow in Georgia Tech’s School of Chemical and Biomolecular Engineering. “We were able to go from milligram-scale powders all the way to prototype membrane modules in commercial form factors that were challenged with real crude oil – it was fantastic to see this innovation pipeline in action.”
ExxonMobil’s relationship with Georgia Tech goes back nearly 15 years and has produced innovations in other separation technologies, including a new carbon-based molecular sieve membrane that could dramatically reduce the energy required to separate a class of hydrocarbon molecules known as alkyl aromatics.
“Through collaboration with strong academic institutions like Georgia Tech and Imperial, we are constantly working to develop the lower-emissions energy solutions of the future," said Vijay Swarup, vice president of research and development at ExxonMobil Research and Engineering Company.
In addition to Finn, Livingston, Lively, and McCool, the paper’s authors include Kirstie Thompson and Ronita Mathias, Georgia Tech graduate students who are co-first authors; Daeok Kim, Jihoon Kim, Irene Bechis, Andrew Tarzia, and Kim Jelfs of Imperial; and Neel Rangnekar, J.R. Johnson, and Scott Hoy of ExxonMobil.
CITATION: Kirstie Thompson, et al., “N-Aryl Linked Spirocyclic Polymers for Membrane Separations of Complex Hydrocarbon Mixtures” (Science 2020). https://science.sciencemag.org/content/369/6501/310
Research News
Georgia Institute of Technology
177 North Avenue
Atlanta, Georgia 30332-0181 USA
Media Relations Contact: John Toon (404-894-6986) (jtoon@gatech.edu)
Writer: John Toon
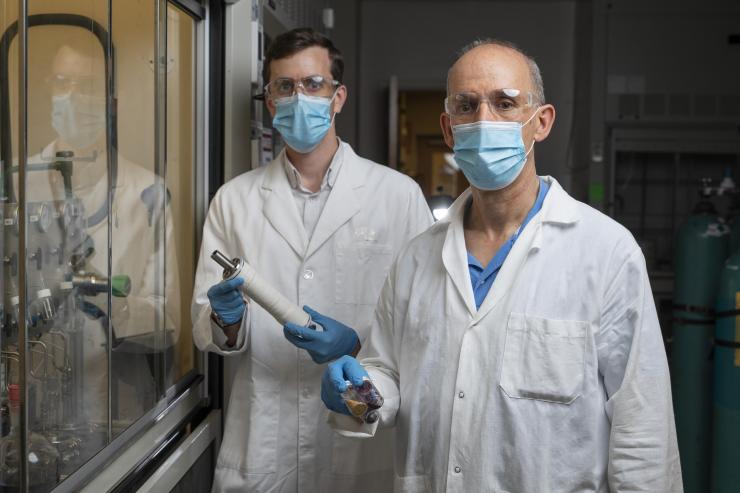
New membrane technology could reduce carbon emissions and energy intensity associated with refining crude oil. Georgia Tech Associate Professor Ryan Lively shows a module containing the new membrane material, while Professor M.G. Finn holds vials containing some of the other polymers used in this study. (Credit: Christopher Moore, Georgia Tech)
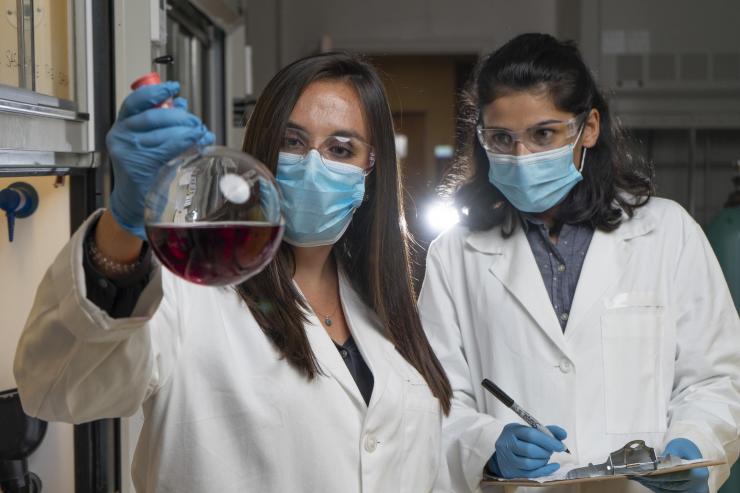
Georgia Tech graduate research assistants Kirstie Thompson and Ronita Mathias with a flask containing polymer materials used to create a new membrane technology that could reduce carbon emissions and energy intensity associated with refining crude oil. (Credit: Christopher Moore, Georgia Tech)
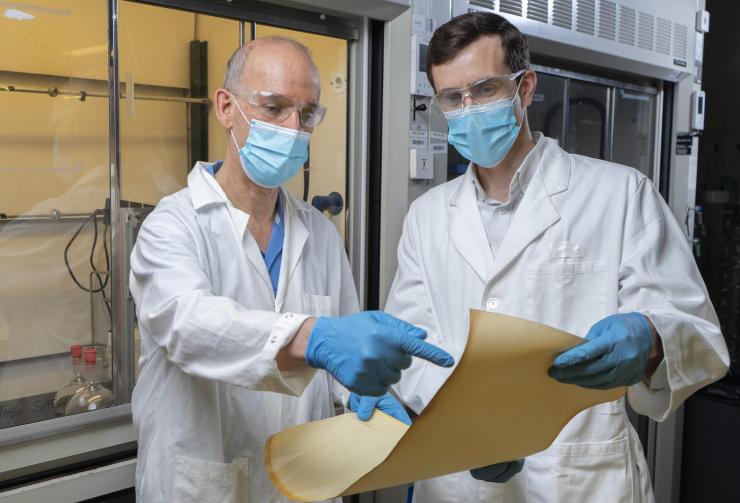
Associate Professor Ryan Lively from Georgia Tech’s School of Chemical and Biomolecular Engineering and Professor M.G. Finn from the School of Chemistry and Biochemistry examine membrane material produced at Imperial College based on a new polymer material. (Credit: Christopher Moore, Georgia Tech)
John Toon
Research News
(404) 894-6986
Ozone Disinfection Could Safely Allow Reuse of Personal Protective Equipment
Jul 09, 2020 — Atlanta, GA
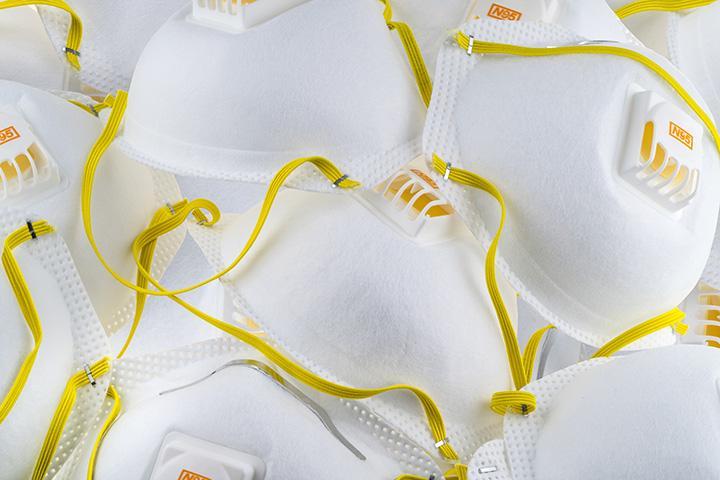
Shortages of personal protective equipment (PPE) can force hospitals and other healthcare facilities to reuse PPE that was intended for a single use. Georgia Tech researchers have studied the use of ozone to disinfect the items. (Credit: Getty Images, not for republication)
A new study shows that ozone gas, a highly reactive chemical composed of three oxygen atoms, could provide a safe means for disinfecting certain types of personal protective equipment that are in high demand for shielding healthcare personnel from Covid-19.
Conducted by researchers at the Georgia Institute of Technology using two pathogens similar to the novel coronavirus, the study found that ozone can inactivate viruses on items such as Tyvek gowns, polycarbonate face shields, goggles, and respirator masks without damaging them — as long as they don’t include stapled-on elastic straps. The study found that the consistency and effectiveness of the ozone treatment depended on maintaining relative humidity of at least 50% in chambers used for disinfection.
“Ozone is one of the friendliest and cleanest ways of deactivating viruses and killing most any pathogen,” said M.G. Finn, chair of Georgia Tech’s School of Chemistry and Biochemistry, who led the study. “It does not leave a residue; it’s easy to generate from atmospheric air, and it’s easy to use from an equipment perspective.”
Findings of the research are described in a paper posted to the medRxiv preprint server and will be submitted to a journal for peer review and publication. Ozone can be produced with inexpensive equipment by exposing oxygen in the atmosphere to ultraviolet light, or through an electrical discharge such as a spark.
During local and regional peaks in coronavirus infection, shortages of personal protective equipment (PPE) can force hospitals and other healthcare facilities to reuse PPE that was intended for a single use. Facilities have used ultraviolet light, vaporized hydrogen peroxide, heat, alcohol and other techniques to disinfect these items, but until recently, there had not been much interest in ozone disinfection, Finn said.
- For more coverage of Georgia Tech’s response to the coronavirus pandemic, please visit our Responding to Covid-19 page.
Ozone is widely used for disinfecting wastewater, purifying drinking water, sanitizing food items, and disinfecting certain types of equipment — even clothing. Ozone disinfection cabinets are commercially available, taking advantage of the oxidizing effects of the gas to kill bacteria and inactivate viruses.
“There was no reason to think it wouldn’t work, but we could find no examples of testing done on a variety of personal protective equipment,” Finn said. “We wanted to contribute to meeting the needs of hospitals and other healthcare organizations to show that this technique could work against pathogens similar to the coronavirus.”
Phil Santangelo, a virologist in the Wallace H. Coulter Department of Biomedical Engineering, recommended two respiratory viruses — influenza A and respiratory syncytial virus (RSV) – as surrogates for coronavirus. The two are known as “enveloped” viruses because, like coronavirus, they are surrounded by a lipid outer membrane. Influenza and RSV are less dangerous than the SARS-CoV-2 coronavirus, allowing the Georgia Tech researchers to study them without high-containment laboratory facilities.
Santangelo, Finn, and their team devised a test procedure in which solutions containing the two viruses were placed onto samples of the PPE materials under study. The solutions were allowed to dry before the samples were placed in a chamber into which ozone was introduced at varying concentrations as low as 20 parts per million. After treatment for different lengths of time, the researchers tested the PPE samples to determine whether or not any of the viruses on the treated surfaces could infect cells grown in the laboratory. The entire test procedure required about a day and a half.
“The protocol we set up reports very sensitively on whether or not the virus could reproduce, and we found that the ozone was very successful in rendering them harmless,” Finn said. “Oxidizing biological samples to a significant extent is enough to inactivate a virus. Either the genetic material or the outer shell of the virus would be damaged enough that it could no longer infect a host cell.”
Loren Williams, a professor in School of Chemistry and Biochemistry, introduced the research team to a manufacturer of ozone disinfection chambers, which allowed evaluation of the equipment using the test protocol. During the test, the researchers learned that having sufficient relative humidity in the chamber — at least 50% — was essential for rapidly inactivating the viruses in a consistent manner.
After subjecting face masks and respirators to ozone disinfection, the team worked with Associate Professor Nga Lee (Sally) Ng from the School of Chemical and Biomolecular Engineering and the School of Earth and Atmospheric Sciences to evaluate the filtration capabilities of the items. The ozone treatment didn’t appear to negatively affect the N-95 filtration material.
But it did damage the elastic materials used to hold the masks in place. While the elastic headbands could be removed from the masks during ozone disinfection, removing and replacing them on a large scale may make the treatment technique impractical. Otherwise, however, ozone may offer an alternative technique for disinfecting other types of PPE.
“Ozone would be a viable method for hospitals and other organizations to disinfect garments, goggles, and gloves,” Finn added. “It is inexpensive to produce, and we hope that by sharing information about what we’ve found, healthcare facilities will be able to consider it as an option, particularly in low-resource areas of the world.”
Beyond those already mentioned, the research involved Emmeline Blanchard from the Wallace H. Coulter Department of Biomedical Engineering at Georgia Tech and Emory University; Justin Lawrence, Taekyu Joo, and Britney Schmidt from the Georgia Tech School of Earth and Atmospheric Sciences; Minghao Xu from the Georgia Tech School of Chemistry and Biochemistry; and Jeffrey Noble from the Parker Petit Institute for Bioengineering and Bioscience.
Research News
Georgia Institute of Technology
177 North Avenue
Atlanta, Georgia 30332-0181 USA
Media Relations Contact: John Toon (404-894-6986) (jtoon@gatech.edu)
Writer: John Toon
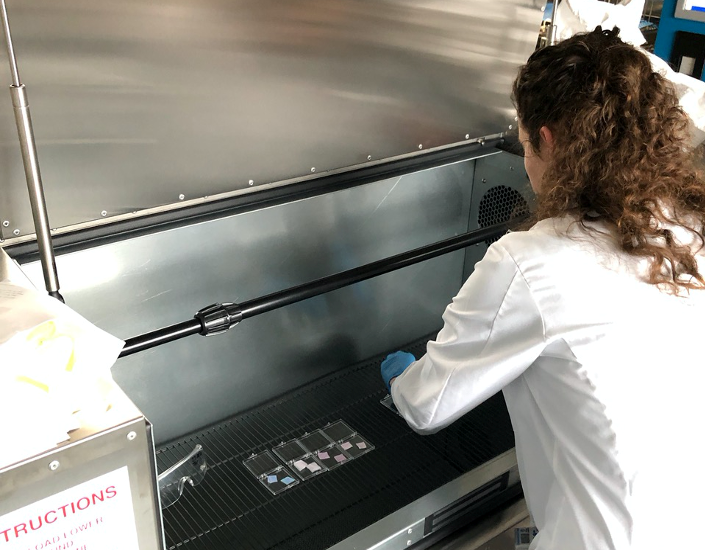
Researcher Emmeline Blanchard from the Wallace H. Coulter Department of Biomedical Engineering at Georgia Tech and Emory University places items into an ozone disinfection chamber for testing. (Photo: Georgia Tech)
John Toon
Research News
(404) 894-6986
Redesigning Hand Sanitizer and Donating 7,000 Gallons to Fight Covid-19
Jun 16, 2020 — Atlanta, GA
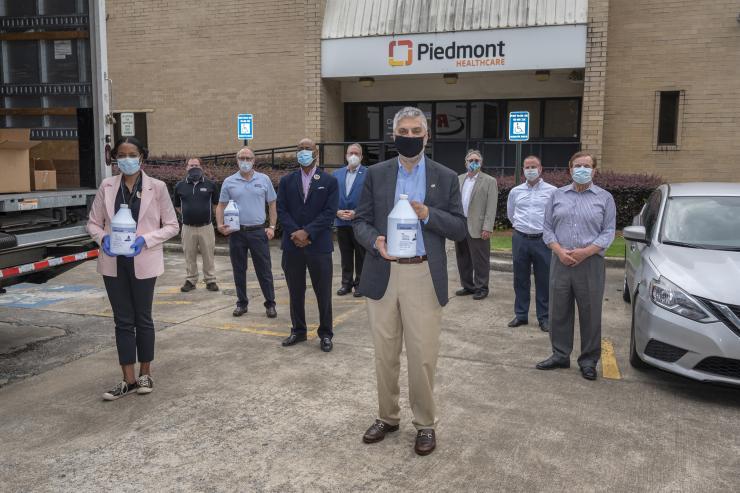
Here, many participants in the effort that re-made hand sanitizer using fuel-grade ethanol on its first day of delivery. The volunteers will donate 7,000 gallons of Han-I-Size White and Gold to hospitals and nursing homes across the country. In front, Dr. Chaouki Abdallah, Executive Vice President for Research, congratulates the group on its success.
So many people Seth Marder spoke to didn’t see the hand sanitizer crisis brewing. The country was going to run dangerously short if someone did not act urgently.
The professor at the Georgia Institute of Technology rallied colleagues and partners around the cause in March, and by early June, they had replaced a key component of hand sanitizer, created a new supply chain, and initiated their own donation of 7,000 gallons of a newly designed sanitizer to medical facilities.
Its name: Han-I-Size White & Gold, named for the colors of Georgia Tech. The new supply chain also may ensure that hand sanitizer producers across the country do not run out of the main active ingredient, alcohol, but the team’s path to success was a stony labyrinth.
“This project was on life support so many times because people did not understand how severe this shortage was going to be,” said Marder, a Regents Professor in Georgia Tech’s School of Chemistry and Biochemistry. “I called hospitals and institutions to assess the need and heard the same thing over and over: ‘No, we just got a delivery. We have no need. You’re wasting your time.’”
Marder was not. Contacts at major chemical suppliers of hand sanitizer ingredients said that a critical shortage of alcohol, particularly the one usually in hand sanitizer, isopropanol, was coming.
“Isopropanol plants in the U.S. were running at full capacity and still didn’t have enough. People were using pharmaceutical-grade ethanol now, too, but it was also in short supply. We weren’t going to have enough of either; I mean the whole United States was running low,” Marder said.
Clean hands cabal
Marder hastily drafted Chris Luettgen, a professor of practice in Georgia Tech’s School of Chemical and Biomolecular Engineering, George White, interim vice president of Georgia Tech’s Office of Industry Collaboration, and Atif Dabdoub, a Georgia Tech alumnus and owner of a local chemical company, Unichem Technologies, Inc.
To the three chemists and the business professional, it seemed simple: Mix alcohol with water, peroxide, and the moisturizer glycerin then bottle and ship it. That bubble burst quickly.
Luettgen, who had worked in the consumer products industry for 25 years at Kimberly-Clark Corporation and knew how to take products to market, had to plow through constant unexpected supply chain barriers and bureaucracy while White forged connections between companies. Neither the supply chain nor the business relationships had existed before, and the teams’ phones stayed glued to their ears night and day as they created them from scratch.
“When I worked for Kimberly-Clark, getting a new product out would take the company nine to 18 months, and the three of us had to get this done in weeks. The demand was there, and people were getting sick in some cases from lack of sanitizing. We felt speed was necessary to meet the growing demand. Seth told me to push this across the goal line, and I put everything into it,” Luettgen said.
“Georgia Tech is about the power to convene. Companies and stakeholders are eager to come to the table here to make things happen,” White said about forging new business ties. “Not everyone has that incredible recognition as a problem solver with the brainpower amassed here.”
Stinking of gin
Purchasing truckloads of alcohol was priority one.
Boutique liquor distilleries in Georgia were already converting to sanitizer ethyl alcohol production, but output was nowhere near enough to meet demand. ExxonMobil connected the team with Eco-Energy, a company that handles fuel-grade ethanol as a gasoline additive.
“The amount of ethanol that’s made for fuel in the U.S. is 1,500 times the amount of the isopropanol made. They could drain off about 1 percent of what is used for fuel and double or triple the amount of alcohol available for hand sanitizer in this country. And the fuel companies wouldn’t even notice it was gone, especially since hardly anyone was driving anymore,” Marder said.
But then prospective hand sanitizer distributors crimped their noses at that ethanol, saying it smelled odd.
“I thought, ‘This has the makings of a screenplay.’ I asked the distributor if we could come over to smell a sample for ourselves,” White said. “It needed a little love.”
Eco-Fuels produced the highly refined ethanol and then processed it through carbon filtration to increase purity and reduce odor. Atlanta-based chemical manufacturer, Momar, Inc., oversaw production, packaging, and distribution of Han-I-Size White & Gold.
The Georgia Tech team garnered funding through a donation from insurer Aflac Incorporated allocated through the Global Center for Medical Innovation (GCMI), a Georgia Tech affiliated non-profit organization that guides new experimental medical solutions to market. Aflac’s gift of $2 million through GCMI has also expedited the development, production, and purchase of other PPE to donate to health care workers.
In addition, GCMI helped guide the hand sanitizer through regulatory processes and to market. The U.S. Food and Drug Administration was also aware of the dire shortage of alcohol for sanitizer and issued waivers for the pandemic to allow for use of ethanol without having to meet usual specifications.
Water, water everywhere
Arkema, Inc. donated hydrogen peroxide, which was delivered to PSG Functional Materials, which mixed and packaged the product then shipped with no delivery fee to Atlanta. Though water is ubiquitous, hand sanitizer requires purified water, and the Coca-Cola Company donated a tanker truck of it just when White was pondering desperate measures.
“If I have to get a truck to go pick up water and drive it, I’ll do it myself,” he said.
Finally, the first few hundred gallons of donated Han-I-Size White & Gold rolled into Piedmont Healthcare in Atlanta and Brightmoor Nursing Center in Griffin, Georgia, in the second week of June 2020.
GCMI is facilitating donations of the 7,000 gallons nationwide. Separate from the Aflac-financed donations, Momar will continue to manufacture the new hand sanitizing formula commercially to include in its regular product lineup, and Georgia Tech will be able to purchase it at a reduced rate to help protect researchers now returning to their labs.
The new supply chain, the first of its kind, of “waiver-grade” ethanol has given hand sanitizer producers across the country a new opportunity to re-supply America.
“Hopefully, we helped solved a national need,” Luettgen said.
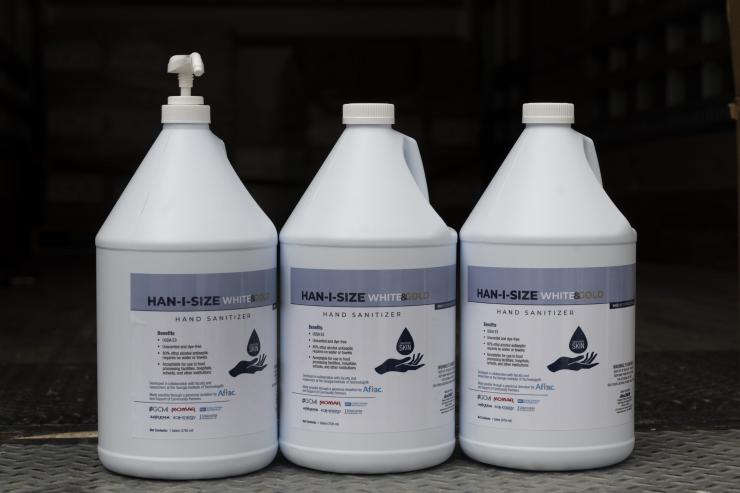
Hand sanitizer was about to run dangerously low across the U.S. but charitable volunteers from Georgia Tech rescued it by turning to fuel-grade ethanol and a special FDA waiver. Then they donated 7,000 gallons to hospitals and nursing homes. Credit: Georgia Tech / Christopher Moore
Emory and Georgia Tech Create Barrier Protection Devices for Use During COVID-19
May 13, 2020 — Atlanta, GA
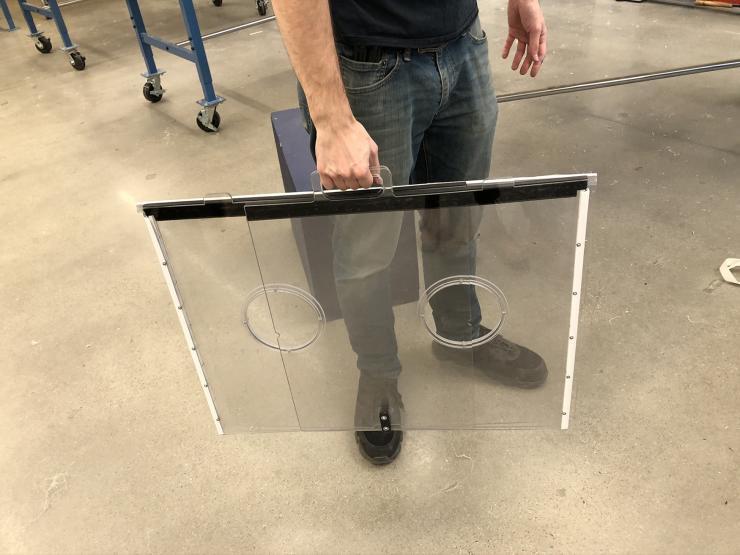
The barrier protection devices can be folded for easy portability and storage at healthcare facilities. (Credit: Georgia Tech)
Medical staff treating patients on the front lines of the COVID-19 pandemic come face to face daily with the risk of exposure to the virus. Among the riskiest moments are inserting and removing breathing tubes, procedures that create a spray of respiratory droplets.
Now, two Atlanta universities have created barrier protection devices designed to contain that droplet spray and aerosol with a goal of reducing the risk of disease transmission.
Made of clear polycarbonate material, the four-sided box is placed on a bed over the patient’s head and shoulders. Protected hand openings allow physicians or other health care personnel to reach into the box to perform procedures such as intubating a patient who needs to be placed on a ventilator.
“Intubation and extubation require a physician to work in extremely close proximity to a patient while simultaneously performing procedures known to generate a large amount of potentially infectious droplets,” said Cinnamon Sullivan, M.D., assistant professor of anesthesiology, Emory University School of Medicine and the director of Global Health Anesthesiology at Emory University Hospital. “The goal of this box is to block, to a large extent, the amount of droplets being aerosolized and serve as one more layer of protection in addition to our personal protective equipment (PPE).”
For more coverage of Georgia Tech’s response to the coronavirus pandemic, please visit our Responding to COVID-19 page.
In recent weeks, a cross-disciplinary team that included anesthesiologists and other physician specialists from Emory University and engineers from the Georgia Institute of Technology has worked quickly prototyping several devices, which were adapted from a basic design distributed widely throughout the medical community as the COVID-19 outbreak grew.
Two primary designs emerged from the effort. One of these devices is a fold-flat box, and the other device is a C-shaped frame. Both provide similar functionalities and are designed for dynamic hospital environments, such as in the emergency department.
The box that can be folded flat when not in use also has a handle to enable easier transportation and includes more safety measures designed to protect clinicians from aerosols escaping through the access holes. These new features were critical to achieving a box that could be used without taking up as much space.
“The medical team that performs intubations moves from unit to unit where we’re needed, so the portability of this design is essential to making it work in actual patient care situations,” said Jeremy Collins, MBChB, FRCA, associate professor of anesthesiology and executive vice chair of anesthesia at Emory. “As well as protecting the anesthesia team, containment of aerosol and droplets generated can minimize contamination to the whole operating room and surrounding corridors.”
The overall goal of the project is to improve protection for medical staff as they work closely with COVID-19 patients, explained Christopher Saldana, associate professor in Georgia Tech’s George W. Woodruff School of Mechanical Engineering. “The goal is to shroud the patient and allow the clinicians to do the necessary procedures while adding an additional barrier from potential exposure,” he said.
The box also helps shield personal protective equipment (PPE) from contamination, potentially helping to maintain supplies.
“A need for such a box was identified during daily meetings with leaders of Emory departments responding to the COVID-19 emergency,” said Susan Margulies, chair of the Wallace H. Coulter Department of Biomedical Engineering that is shared by Georgia Tech and Emory. From the meeting, Margulies identified problems that might be addressed by Georgia Tech researchers.
“My role is to think about how the expertise at Georgia Tech can be brought to bear on the needs of the medical community,” she said. “As a department truly embedded on both campuses, this collaboration gives us the opportunity to create a direct relationship between the problems and the solutions.”
Margulies brought the aerosol containment issue to Sam Graham, chair of the Woodruff School of Mechanical Engineering, and Saldana, whose research focuses on manufacturing and materials. Saldana listened to the problem and worked with Margulies to quickly develop a concept that could be evaluated, based on a design used in Asia.
Based on the initial concept, Saldana and graduate student Kentez Craig quickly built two prototypes and sent them to Emory for Sullivan, Collins and others to inspect and check whether the size of the box would work in an operating room environment. “Emory told us they really needed them,” Saldana said. “They showed us how this design would be used in practice and we talked about iterations.”
Sullivan and Collins immediately identified the need to make the devices more portable, as well as address how the access holes could be better closed off to prevent aerosols from escaping during use.
Since these developments, a team of graduate students, including Jaime Berez and Maxwell Praniewicz, quickly designed the final prototypes of the fold-flat box and the C-shaped frame. Review and testing of the C-shaped frame was completed with Russell Gore, M.D., an adjunct associate professor in the Wallace H. Coulter Department of Biomedical Engineering, Adam Klein, M.D., a professor in the Department of Otolaryngology at Emory University and David Wright, M.D., a professor and chair of the Department of Emergency Medicine at Emory University. To produce these designs, Siemens Corporation joined the team to lead the production of prototypes; Barry Powell and James Washburn at Siemens implemented an industrial manual assembly process with additional support from Georgia Tech’s Montgomery Machining Mall and the Georgia Tech Research Institute’s Machine Services.
The boxes and frames are made from polycarbonate, a clear rigid material. The material was cut in Georgia Tech’s Flowers Invention Studio with a water-jet machine. A laser device was used to cut the hand holes. “You might need some specialized equipment, but most people could use general shop equipment to produce these,” Saldana said.
People who’ve worked in a research laboratory will recognize the concept behind the devices. “This is a lot like a glovebox that is used in many laboratories to separate laboratory technician from hazardous materials or environments inside the box or frame,” Saldana said. “The technician places their hands and arms into the gloves, allowing them to work separate from what’s inside.”
Unlike the face shields and respirators that are in such high demand, the barrier protection devices will be needed only in small quantities to shield clinicians during the specific procedure. Saldana says hospitals potentially could find it useful in emergency departments, intensive care units and operating rooms.
“We hope these barrier protection devices have utility beyond this outbreak,” Sullivan said. “They may be able to be used for any aerosolized disease, and with the modifications we are making, it could be taken to areas with fewer PPE resources both here in the U.S. and overseas.”
Research News
Georgia Institute of Technology
177 North Avenue
Atlanta, Georgia 30332-0181 USA
Media Relations Contacts: Georgia Tech – John Toon (jtoon@gatech.edu); Emory Healthcare – Josh Brown (joshua.g.brown@emoryhealthcare.org).
Writer: Joshua Brown
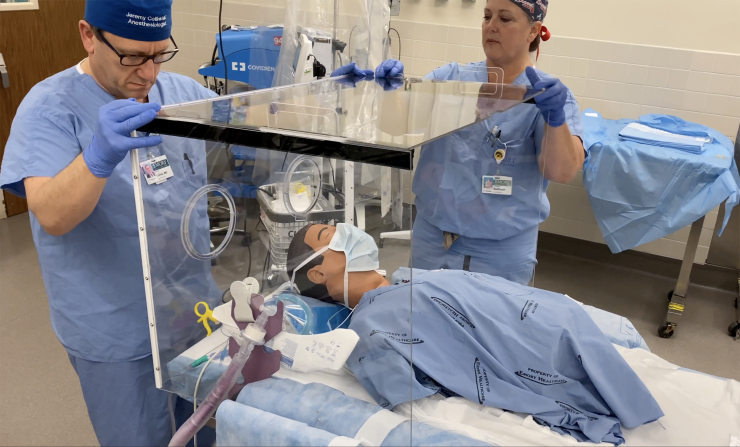
Jeremy Collins and Cinnamon Sullivan demonstrate a barrier protection device designed for use curing the COVID-19 pandemic. Collins is associate professor of anesthesiology and executive vice chair of anesthesia at Emory and Sullivan is assistant professor of anesthesiology, Emory University School of Medicine and the director of Global Health Anesthesiology at Emory University Hospital. (Credit: Emory Healthcare)
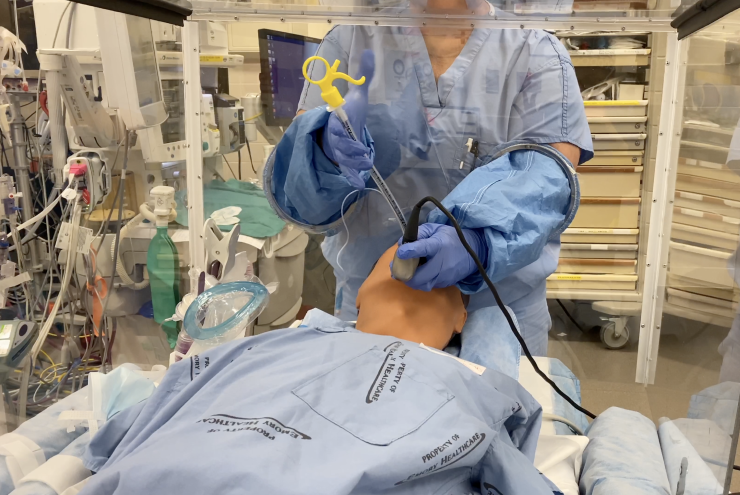
A clinician demonstrates how a barrier protection device allows procedures to be done while providing protection against aerosols from a COVID-19 patient. (Credit: Emory Healthcare)
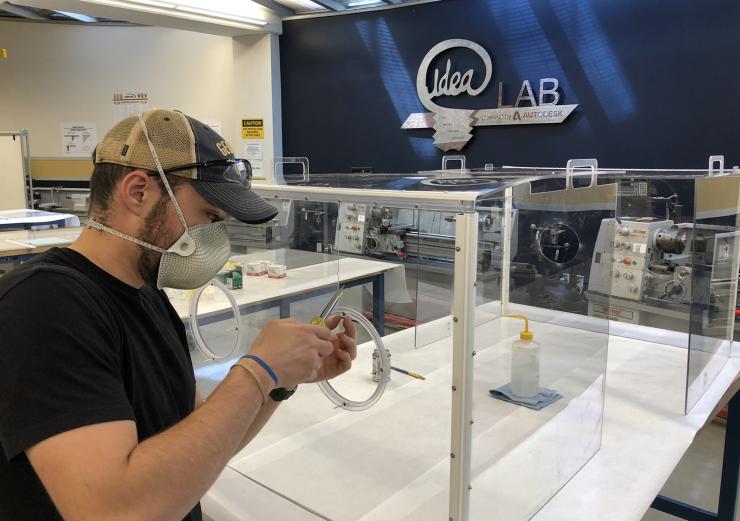
The barrier protection devices were designed and fabricated at the Georgia Institute of Technology based on feedback from clinicians at Emory Healthcare. (Credit: Georgia Tech)
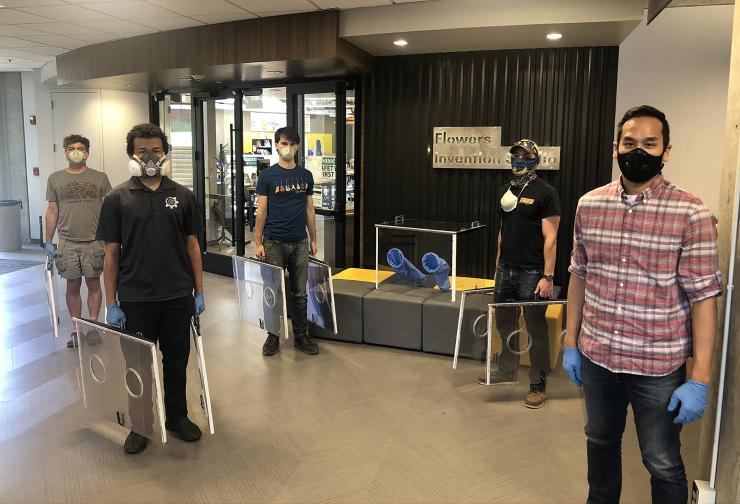
A team of Georgia Tech faculty and students created prototype barrier protection devices (Credit: Georgia Tech)
John Toon
Research News
(404) 894-6986
Do-It-Yourself Medical Devices and Protective Gear Fuel Battle Against COVID-19
Mar 23, 2020 — Atlanta, GA
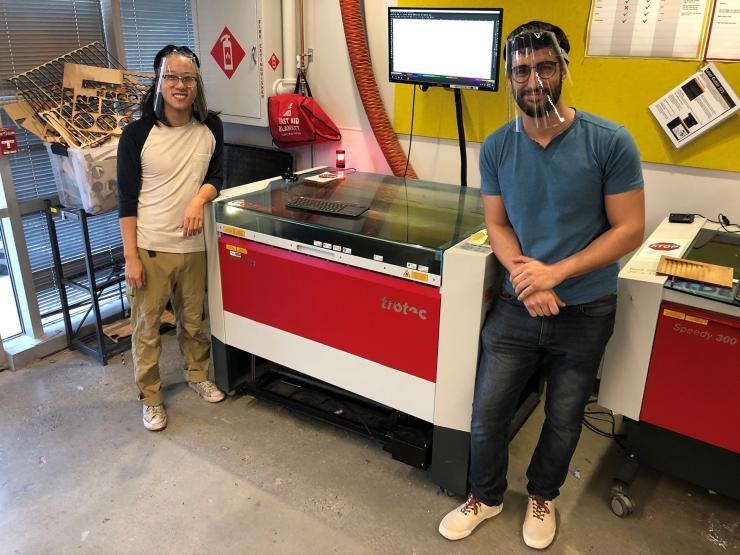
To help meet the need for personal protective equipment (PPE) for health care workers, Georgia Tech has designed and is producing face shields. Shown is a laser cutting machine used to create frames for the shields.
It’s a race against time that some participants liken to Apollo 13, the stricken NASA spacecraft for which engineers improvised an air purification system from available parts to get three astronauts back from the moon.
In this case, however, the race is to improvise ventilators, face shields, respirators, surgical gowns, disinfectant wipes, and other healthcare gear to help the hundreds of thousands of people expected to swamp hospitals with waves of critical COVID-19 illness over the next several weeks. The demand for ventilators alone could be four times more than already overwhelmed hospitals can provide.
Using 3D-printed parts, plastic-lined tablecloths intended for birthday parties, laser-cut gears, and similar substitutions, a research team from universities on two continents is racing to develop “do-it-yourself” healthcare gear that can be assembled where it’s needed from components available locally. Team members figure they have about two weeks to get the designs right and share them with anyone who can help with the needs.
“We’re trying to figure out how to get these things to scale in the time we have,” said Shannon Yee, an associate professor in Georgia Tech’s George W. Woodruff School of Mechanical Engineering who’s working on the ventilator issue with a half-dozen colleagues at Georgia Tech and other universities. “We are looking at producing things very quickly and this is where having contacts with mature manufacturing sources is going to help.”
- Georgia Tech has established a Rapid Response website to identify needs for personal protective equipment and potential collaborations.
Supplying Face Shields to the Medical Community
The Wallace H. Coulter Department of Biomedical Engineering at Emory and Georgia Tech serves as a bridge between healthcare needs and the broad technical know-how at Georgia Tech, and Georgia Tech researchers are talking regularly with hospital systems to discuss their needs. So far, hand sanitizer, disinfectant wipes, face shields, respirator masks, and ventilators have been identified as critical needs. Using resources of the Flowers Invention Studio – such as 3D printing – the group has already produced 1,000 face shields and is preparing to fabricate thousands more in the form of kits that hospitals can assemble.
"With the significant challenges on our supply chain, we need strategies to provide personal protective equipment (PPE) for healthcare staff," said Dr. Charles Brown, CEO of Physician Enterprise at Piedmont Healthcare. "We have mechanisms in place to develop ideas and are working with Georgia Tech and the Global Center for Medical Innovation (GCMI) to advance them to what we can use."
Georgia Tech faculty members, students and GCMI worked on multiple face shield designs, talking with clinicians at Children’s Healthcare of Atlanta, Emory Healthcare and Piedmont to evaluate and iterate. The result was two different designs intended for specific uses in hospital facilities, where face shields protect clinicians from splashes and help extend the life of soft respirators intended to filter out virus particles.
“The team has worked hard to identify materials suppliers and define simple and scalable solutions to meet this challenge,” said Sam Graham, chair of the Woodruff School of Mechanical Engineering. “We are fortunate to have partners ready to team up with us to help address some of the shortfalls in medical equipment that hospitals are experiencing."
To scale up fabrication beyond the Georgia Tech campus, the team focused on simple designs that could be shared with and produced by individuals with access to a makerspace – and major manufacturers with injection molding capabilities. The team plans to make the designs available for anyone with laser cutting or 3D printing capabilities.
“Initially we were just thinking about meeting the needs of Atlanta, but cities everywhere need them,” said Saad Bhamla, an assistant professor in the School of Chemical and Biomolecular Engineering who specializes in “frugal science” – creating inexpensive lab devices. “We have created great models that can be used to create a pipeline of instructions that others can use. The face shields will set the stage for other device models as they become available.”
The group is leveraging Georgia Tech contacts with companies to identify suppliers for alternative materials that can go into their “Apollo 13” devices. Team members, including Christopher Saldana, an associate professor in the Woodruff School, are working with GCMI on those issues, using equipment in Georgia Tech’s maker spaces and elsewhere.
"The Georgia Tech mechanical engineering team is working to modify open source face shield designs so they can be manufactured in high volumes for the rapid response environment that COVID-19 requires,” said Christopher Saldana, an associate professor in the Woodruff School. “Our team has modified these designs using a range of product and process optimization methods, including removing certain features and standardizing tool use. By working on cross-functional and cross-disciplinary teams and directly involving healthcare practitioners and high-volume manufacturers, we will be able to respond to this effort at the scale and speed required."
Bringing Georgia Tech’s expertise together to address the challenges – and develop collaborations – has been done behind the scenes by people like Sherry Farrugia, chief operating and strategy officer for the Children’s Healthcare of Atlanta Pediatric Technology Center.
“Serving as kind of a chief strategy officer, my work is to help bridge the gaps, focus the teams, rally the troops, and make critical connections,” she said. “Doing this requires a deep knowledge of who’s doing what on campus, as well as a strong network in the private sector.”
The Supply Chain Challenge
The team is launching a website (www.research.gatech.edu/rapid-response) to both quantify the needs for face shields and solicit supplies of materials. Because the world’s supply chains are unable to ship conventional PPE components, they are looking for alternatives that may not now be part of that production.
The challenge is that everyone is scrambling to find equipment and materials in an international supply chain that has already been depleted by months-long demands from countries that dealt with the virus earlier: China, Italy and South Korea. As the healthcare demands ramp up in the United States, hospitals will have to be more creative in meeting the needs that their traditional sources may not be able to supply.
"Countries on the trailing end of the pandemic are facing supply chain issues that countries with earlier pandemics didn't have to face," said Michael O'Toole, Executive Director of Quality Improvement at Piedmont and a Georgia Tech engineering graduate. "We've got to get these supplies, and its a critical need already. If we can't get them from commercial or government sources, we're going to have to make them ourselves."
With significant efforts going into design of locally sourced equipment, expertise on medical device prototyping and approval is needed. That is coming from a network of alumni and local companies and GCMI, a Georgia Tech-affiliated organization that works with device manufacturers around the world to translate designs into devices that can be manufactured quickly and cost effectively.
“The goal right now is to develop solutions that can be sourced locally and that we can produce now,” said Tiffany Wilson, GCMI’s CEO. “We are working with Georgia Tech and others on how we can suggest modifying the designs to optimize them for the current environment. We are helping make sure designs are clinically validated with an eye toward scalability.”
Beyond its experience with medical devices, GCMI is also helping source materials and components, and working with regulators at the FDA to help reduce risks in the responses.
“There have been changes in some of the standards and new guidance from the FDA to enable faster production to open up the supply chain to get more masks and respirators into the market,” Wilson said. “There are still levels of control and risk mitigations strategies that we need to focus on. We’re staying on top of those changes.”
Research on Possible Solutions for Other Shortages
While the face shield is the most mature project the team is developing, researchers are also looking at other needs of the medical community. Among them are ventilators, disinfecting wipes, and respirators.
An example of an Apollo 13 project may be ventilators that are used to help critically ill patients breathe. Traditional equipment makers are working as fast as they can, but that may not be fast enough. To achieve a globally scalable makeshift ventilator will require minimizing the number of parts and thinking about mechanical simplicity, Yee said.
Leon Williams, head of the Centre for Competitive Creative Design at Cranfield University, is working with Georgia Tech researchers to create a makeshift ventilator based on the bag-valve-mask (BVM) – also known as an Ambu bag – a hand-held mechanical resuscitation device already available at hospitals.
Through a system of laser-cut gears and other components, the preliminary concept would use a simple three-volt motor to compress the bag and push air into the lungs of a critically ill patient. Among the challenges is extending the lifetime of the bags, which are not designed for long-term use.
“We need to understand everything about the ventilators that are already in use,” said Susan Margulies, chair of the Wallace H. Coulter Department of Biomedical Engineering at Georgia Tech and Emory University. “By understanding how everything works, we can modify the design to use the components we can get.”
As with face shields, the group expects to make its plans widely available for other groups to iterate and produce. “There is a lot of activity here that is going to move this forward,” said Devesh Ranjan, associate chair for research in the Woodruff School of Mechanical Engineering, who is coordinating several of the Georgia Tech Rapid-Response projects on campus.
Another identified need is for disinfecting wipes, which seem like a simple enough product: a nonwoven material and a solution based on either alcohol or bleach. The material and solutions seem to be available; the problem is locating the industrial-sized containers to hold them.
“We’ve been looking for containers for the wipes commercially,” said Graham. “What we are finding is that the issue is the containers, but we are looking at other solutions.” He’s working with David Sholl, chair of the School of Chemical and Biomolecular Engineering, to identify potential suppliers.
Respirators, Swabs and Gowns
Protecting healthcare workers from the coronavirus requires a special type of respirator, soft face masks that remove virus particles from the air. Because the virus particles are so small, hundreds of nanometers in diameter, that protection requires high-efficiency filtration materials that until recently were mostly manufactured in China.
“The filters are not being produced at the rates that are needed, so we have been thinking about what we can put together that approximates an N95 filter that’s needed to protect healthcare workers,” said Ryan Lively, an associate professor in the School of Chemical and Biomolecular Engineering. “We need to make something that can be produced out of homemade goods, then verify that it can do the filtering needed.”
Lively has been experimenting with alternatives, such as high-efficiency filtration materials manufactured for HVAC systems that could be sewn inside a fabric pouch. “There are journal papers out there showing filtration materials that are not as good as N95 are still effective at increasing rejection of the virus particles,” he said.
If these work as needed, Lively could produce limited numbers in his lab. “We have estimated that we can produce 700 masks per week using the pilot line that we have for research and repurposing it for cranking out hydrophobic fiber media,” he said. “That won’t solve the problem, but it will help meet a very critical need.”
The swabs used for COVID-19 testing are also in short supply, as are gowns designed to protect healthcare workers. Carson Meredith, director of the Renewable Bioproducts Institute, is tracking down alternative sources from among the many manufacturers who are members of the Georgia Tech interdisciplinary research institute.
“The idea is to take a basic material intended for a different function and transform it into the products that we need,” he said. One example is a material manufactured for party tablecloths - plastic on one side to prevent spills from going through, and paper on the other for festive designs. “We’re looking at whether the machinery that produces those can be rapidly turned into making a temporary gown.”
The research team meets by phone daily to update each other on what’s been done and to share ideas. They follow international Slack channels to know what other similar groups are doing across the U.S. and the world.
They know their prototype production equipment can’t meet the world’s needs, so they’re sharing plans with others who may have capabilities. Ultimately, major manufacturers will catch up, but that could take months – perhaps too long for the expected COVID-19 infection curve.
“The best thing we can do is share that information broadly to try to come up with solutions that use parts that can be sourced locally,” Yee said, referring to the ventilator project. “Simple solutions using motors that people can get anywhere, structures that can be 3D-printed and materials that can be hand-cut with saws may get us through this.”
Article updated March 26, 2020
Research News
Georgia Institute of Technology
177 North Avenue
Atlanta, Georgia 30332-0181 USA
Media Relations Contacts: John Toon (404-894-6986) (jtoon@gatech.edu) or Ben Brumfield (404-272-2780) (ben.brumfield@comm.gatech.edu)
Writer: John Toon
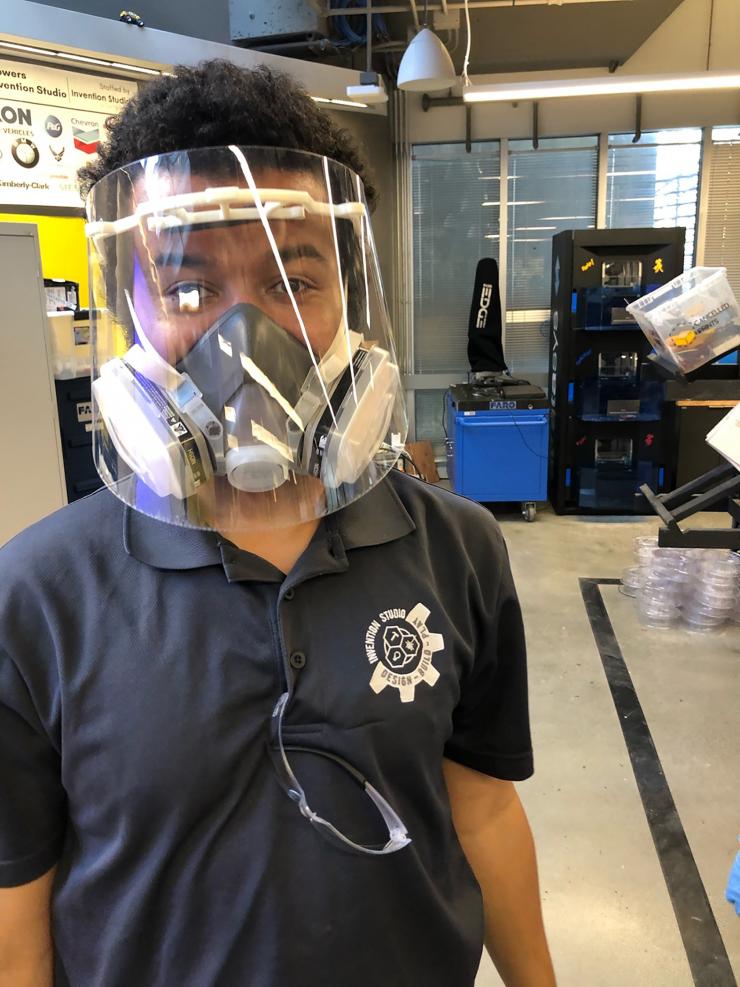
Georgia Tech is helping meet the need for face shields for health care workers.
John Toon
Research News
(404) 894-6986