NASA Research Isn't All Rocket Science
Oct 18, 2019 — Atlanta, GA
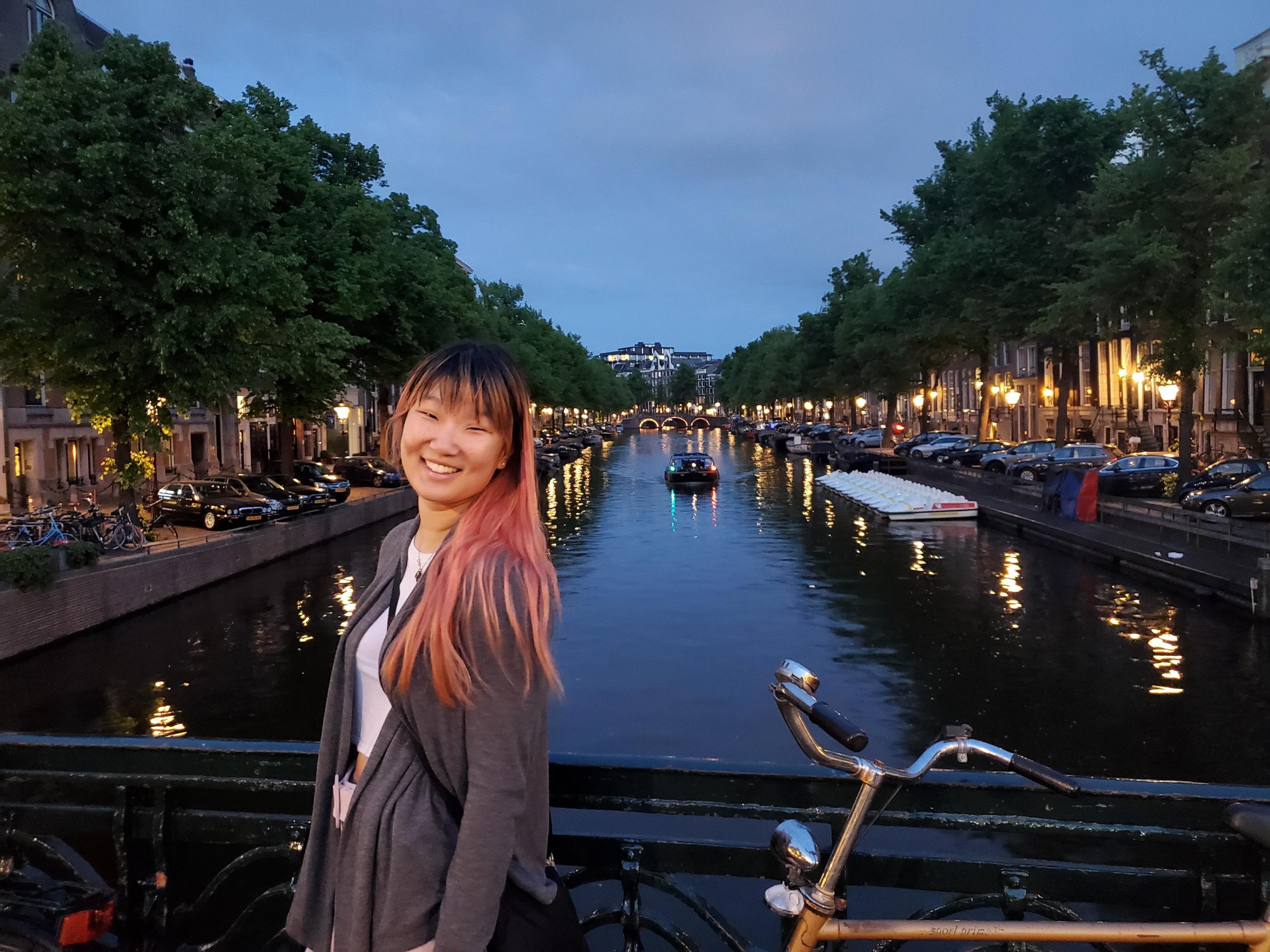
Bioscientists who want to shed light on the origin, evolution, and distribution of life in the universe don’t have to travel very far to do it. In fact, they’re doing it right now at the Petit Institute of Bioengineering and Bioscience at the Georgia Institute of Technology, where multidisciplinary researchers are proving that not all space research is rocket science.
The National Aeronautics and Space Administration, NASA, established in 1958 to lead the United States’ space exploration efforts, started supporting the search for extraterrestrial life the following year with a grant for the development of the ‘Wolf Trap,’ a tool designed to detect microbial life on the surface of another planet. Life beyond Earth hasn’t been found yet, but after 60 years we have a much better idea of what we should be looking for.
The quest continues today, with the aid of investigators at the Petit Institute where the NASA-supported research is not only focused on untangling the roots of life here and elsewhere in the galaxy, but also on protecting the health and safety of the next generation of astronauts who will explore outer space.
Petit Institute researchers are actively engaged in what School of Biological Sciences Professor Frank Rosenzweig calls, “the twin pillars that have supported NASA since its inception – human space exploration and astrobiology, the study of the origins and distribution of life in the universe.”
They are compatible tracks, says Thomas Orlando.
“We’re definitely on a connective path with the people engaged in astrobiology,” declares Orlando, principal investigator for the REVEALS Project (Radiation Effects on Volatiles and Exploration of Asteroids and Lunar Surfaces), a member of SSERVI (Solar System Exploration Research Virtual Institute), formerly the NASA Lunar Science Institute.
REVEALS, based at Georgia Tech, is actually an international multidisciplinary team seeking to develop new materials to detect and shield future astronauts from radiation, and researching the interaction of radiation and micrometeorites with the surfaces of airless bodies, like the Earth’s moon, or an asteroid, or the moons of Mars (Phobos and Deimos). These interactions can either produce molecules such as water, hydrogen and methane relevant to sustaining the presence of humans on the Earth's moon. They can also lead to the formation of molecules relevant to the emergence of life.
“We want to develop material that could be integrated into the spacesuit,” says Orlando, professor in the School of Chemistry and Biochemistry, and adjunct professor in the School of Physics, associate director of the Strategic Energy Institute, and Co-founder and former director of the Center for Space Technology and Research. “Robust material that would be electrically conductive, good with regard to heat transport properties, and very strong mechanically – no added weight, but added value. It’s about improving the tools that we have and changing the way we do things.”
The U.S. has committed to returning people to the moon, and Orlando’s research could help with the success of future missions. To expand this, he is also a co-investigator of HOME, a NASA sponsored Space Technology Research Institute at the University of California Davis that focuses on keeping astronauts and their habitats “alive” for extended human missions. As a chemical physicist, he is also particularly interested in what exploration of the moon’s polar regions might uncover, since they may be the “chemical libraries” of what was available during planet formation.
“We’re very interested in the poles because we know there are organics there,” he says. “It would be useful to know what the organic inventory is, because it’s a pristine environment. It hasn’t seen solar wind, hasn’t seen photons, it’s really cold – 25 Kelvin. It’s chemistry that’s been there a long time. I call it the Hotel California for molecules – once they’re there, they never leave.”
He makes a case for the moon’s polar regions as an excellent laboratory for finding potential clues to the roots of life. Easier to find a needle in a sparse haystack.
Meanwhile, some of Orlando’s Petit Institute NASA-supported colleagues are studying model systems on Earth that may offer hints of how to find life on distant moons. Others, working from different directions, are approaching the elusive golden spike linking prebiotic chemistry with modern biochemistry – the origin of life. Some are studying the evolution of multicellularity. At least one is trying to ensure the healthy vision of astronauts. And a cluster of Petit Institute faculty that are engaged in astrobiological research have attained real literary immortality as comic book heroes – all part of a day’s work when you’re a rock star in your field.
“I am, admittedly, a science geek, and I have been for years,” explains Linda Billings, a consultant to the NASA Astrobiology Program, who spearheaded the graphic history project. “So my heroes and rock stars are scientists, and Georgia Tech has some real rock stars.”
Visionary Thinking
Ross Ethier is interested in what happens to astronauts when they spend long periods of time in space. Specifically, he’s interested in what happens to their vision, because it turns out that 40 to 50 percent of them develop a condition called Space Flight Associated Neuro-ocular Syndrome, or SANS.
“Basically, the back of their eye gets a little bit flatter,” says Ethier, professor in the Wallace H. Coulter Department of Biomedical Engineering at Georgia Tech and Emory University. “The sheath around the optic nerve swells up and we think it has profound effects on the function of the eye.”
Ethier’s team (which includes fellow Petit Institute researcher John Oshinski, associate professor of radiology and imaging sciences at Emory) are using a combination of optical coherence tomography and computer modeling, “to quantify the effects and develop a risk profiling tool. NASA is also interested in countermeasures – could we do something in space to help prevent this from happening? That’s a work in progress.”
SANS is similar to an Earth condition, idiopathic intracranial hypertension. Some of the vision issues in space are cleared up when the astronaut gets back to Earth, “but it isn’t all recovered. It’s not a trifling matter,” Ethier says. “So hopefully we’re contributing to making sure our astronauts can keep great vision when they’re exploring.”
While Ethier and Orlando are both working on projects related directly to astronaut health and safety, most Petit Institute researchers engaged in NASA work have their feet and focus firmly on the ground – or much, much deeper. These are the scientists engaged in different aspects of astrobiology. And three of them – Nick Hud, Frank Rosenzweig, and Loren Williams – have helped chart the course of planetary research as part of the team of lead authors of the NASA Astrobiology Strategy. (Note: Another Georgia Tech researcher, but not affiliated with the Petit Institute, is Britney Schmidt a national leader in astrobiology research from the School of Earth and Atmospheric Sciences, also a lead author of the 2015 document).
“As we were creating this large document I saw an opportunity to put together a team that would be a node of the NASA Astrobiology Institute, that would engage on activities related to the theme of the evolution of complex life,” says Rosenzweig, who was at the University of Montana at the time.
“The areas we’ve been interested in are what we’re calling major transitions,” he added. “There were a lot of people in the evolutionary biology community that were working on different problems and I thought, why not put all those people under one tent and call it an astrobiology institute? And we were real lucky to have a great bunch of curious investigators come on board.”
One of them is Petit Institute researcher Will Ratcliff, associate professor in the School of Biological Sciences, who was named one of the 10 most brilliant people of 2016 by Popular Science magazine for his explorations into the origin of multicellularity by experimentally evolving yeast.
“NASA’s interested in using laboratory models to really try and dial in on key factors that could unlock the potential to evolve increased complexity,” says Ratcliff, whose multidisciplinary team includes fellow Petit Institute researcher Peter Yunker, assistant professor in the School of Physics.
Ratcliff hopes to continue the long-term evolution experiment for the next 30 years, so his snowflake yeast will keep evolving at a rapid pace, growing larger and becoming more complex. So far they’ve gone through more than 800 rounds of selection (about 4,000 generations) and have evolved yeast that are now about 10,000 times larger than their simpler multicellular ancestor, with novel genetically-regulated development. “They’re actually macroscopic,” says Ratcliff. “You can now see individuals with the naked eye.”
But there are costs associated with size, “a soft constraint,” Ratcliff says. “If they can’t figure out how to get around that, it means they won’t be able to succeed, so they have to get around that constraint somehow, which means they have to evolve new, tougher bodies, and a lot of our research is trying to figure out how they do that.”
Since he can’t go to the moons of Jupiter and Saturn, or further, to observe how life might evolve, Ratcliff is doing it at Georgia Tech.
“But I would be completely shocked if life was a really rare thing in the universe,” he says. “The problem is, the universe is so spread out that it’s very difficult to encounter anything else, biotic or abiotic. So we have to think of new tools for studying evolutionary or ecological or even chemical transitions. I think our best bet for rapid progress is going to be a well-controlled laboratory system.”
Deep Dive Into Space
Jennifer Glass, who loves to do field work, doesn’t expect to visit Titan, Saturn’s exotic icy moon. But working in her lab at Georgia Tech, she’s trying to find clues of what may lie beneath Titan’s frozen surface. At the very least, she’s developing an understanding of what we should be looking for when NASA gets there in 2034 with the Dragonfly mission to get an intimate look at the moon’s nitrogen-based atmosphere (like Earth).
Unlike Earth, Titan’s clouds and rain are made of methane. Other organics fall like snow and on the moon’s surface, which has the kind of organic chemistry that might have sparked life on Earth.
“Icy moons like Titan, or Jupiter’s moon, Europa, have interesting, potentially habitable ecosystems called hydrates, where waters are arranged around a gas molecule,” says Glass, associate professor in the School of Earth and Atmospheric Sciences. “We know life needs liquid water on Earth to survive, so we’re wondering, is it possible that bacteria can actually modulate the stability of those methane hydrates?”
So she’s leading an interdisciplinary team of Georgia Tech researchers (geo-microbiologists, biochemists, and geo-engineers) on a NASA Exobiology Program project called Microbial Interactions with Methane Clathrate: Implications for Habitability of Icy Moons. It’s a search for DNA blueprints of potential clathrate-binding proteins. The investigators will reproduce those proteins in a lab and test their impact on methane clathrate properties.
“We’re looking into the DNA that we find, deep, deep below the sea floor – genes that have never been studied in a lab before – and expressing them in a lab, then testing them in simulated deep ecosystems,” says Glass, who is collaborating on the NASA project with (among others) Petit Institute researcher Raquel Lieberman, professor in the School of Chemistry and Biochemistry. Lieberman’s lab is producing the proteins that Glass and her team identified from their ocean floor genomes.
Glass approached Lieberman about the astrobiology project last year, knowing her reputation as an expert in expressing and purifying proteins of all shapes and sizes.
“The proteins we’re producing in my lab have the potential to bind ice crystals that contain methane gas,” says Lieberman.
The motivation is to understand how these unique microbes, these bacteria, “are making a living in these deep, remote ecosystems,” says Glass. “They’ve never been cultivated in a lab before, so this is how we can explore, using biochemistry approaches. We’re interested in these deep sediment, deep biosphere ecosystems because they are, in a way, analogs to icy moons like Europa and Titan.”
(Note: Amanda Stockton in the School of Chemistry, who isn't a Petit Institute researcher, is funded by NASA as part of a mission to Europa in a search for biomolecules).
The Golden Spike
Nick Hud uses the golden spike analogy to describe how he and his longtime colleague Loren Williams are approaching their search for the origins of life on Earth. In the 19th century, he explains, “there were teams working from the west coast and the east coast, building the railroad, with the idea of a continuous link. They would meet somewhere to complete the link and drive home the golden spike. What we want to know is how life started and how it evolved to what it is today.”
Hud, Regents Professor in the School of Chemistry and Biochemistry, is associate director of the Petit Institute, and director of the NSF Center for Chemical Evolution (CCE). Williams, a founding faculty member of the Petit Institute, and also a professor of chemistry and biochemistry, is director of the Center for the Origin of Life (COOL).
Hud’s team takes a bottom up approach that begins with geology and chemistry and an understanding of the formation of the first polymers of life from a prebiotic age. Williams’ top down approach starts with biology, genetics, and looking back in time, like counting back the rings in the cutting of a large tree. In Williams’ view, “the essence of biology is the persistence of fragile molecules.”
Like railroads being constructed from opposite ends of the country, Hud and Williams plan to meet somewhere in between, their approaches converging on the coevolution of the biopolymers of life. The golden spike.
“We can look at elements within a cell and understand in some cases why something would have evolved, or what evolved before, in terms of different parts in the cell, different functions of the cell, and organisms,” Hud says. “We can get back pretty far, but we may never work our way all the way back in time, because life has evolved and changed so much that we lose some traces. On the other side is prebiotic chemistry. We can look there and ask, ‘can we go forward in time,’ from the chemistry we’d expect would have been on the early Earth, go forward to get to molecules that look like, or may be identical to, what we still see in life today.”
It’s wrapped within Hud’s proto-RNA hypothesis, as he and his colleagues search for ancient molecules with the ability to self-assemble into RNA- or protein-like polymers, which would represent a major advancement in the field of molecular self-assembly (persistent molecules).
“We’re like molecular archaeologists,” says Williams.
The two researchers, Hud and Williams, have been working closely, though from opposite angles, for years. Hud is an affiliate member of COOL (a NASA-funded research center launched in 2018), and Williams is a scientific collaborator in CCE (an NSF/NASA center formed in 2007). In fact, the CCE team includes a number of other Petit Institute researchers: Martha Grover, Thomas Orlando, Facundo Fernandez, Stefan France.
Most of them are now comic book heroes thanks to the NASA Astrobiology graphic history series (Issue No. 7, released over the summer, Astrobiology: The Story of our Search for Life in the Universe). Hud, Williams, Grover, Fernandez, France, and Bradley Burcar (research scientist in Hud’s lab) are given the comic book treatment, which is appropriate according to Williams (referring specifically to he and his old friend Hud), who quips, “a comic book is the optimum medium by which we should be immortalized.”
The Next Generation
NASA has been supporting Petit Institute researchers for years as they search for the secrets of life in laboratories, deep under the ocean, or on distant planets and moons. And the space agency is helping to ensure the success of future missions by helping to develop the next generation of space scientists at Tech.
Several undergraduate students in the Coulter Department of Biomedical Engineering have worked as NASA interns over the past several years. Right now, fourth-year student Julia Woodall is interning at the Kennedy Space Center, where she’s studying the mechanical effects of microgravity on cells.
“I conduct research on cell mechanics, specifically on red blood cell fragmentation in clotting disorders,” explains Woodall, who says she actually became interested in microgravity through her work through the Astronaut Scholarship Foundation.
Micah Schaible’s research, meanwhile, is supported through the NASA Postdoctoral Program (NPP), which allows promising Ph.D. scientists and engineers to perform research on problems mostly of their own choosing, but compatible with the research interests of the NASA Astrobiology Program.
Schaible, who earned his Ph.D. in applied physics at the University of Virginia (UVA), is examining the potential effects of charged dust grains on humans in space as part of Thomas Orlando’s NASA SSERVI REVEALS team, in the EPICS (Electron and Photon Induced Chemistry on Surfaces) lab at Georgia Tech. He got involved in space science by accident, after walking into the first lab at UVA that had funding for a grad student.
“They happened to be doing space science, and I saw all these hoses hanging everywhere, wires going every which way – kind of like the EPICS lab – and I thought, ‘I really like this environment,’” Schaible says. “Let’s figure out what all this stuff does.”
Today he’s running his own experiment, collaborating with SSERVI teams at the University of Colorado-Boulder and the NASA Goddard Space Flight Center, studying how ionizing radiation causes changes to organic molecules and dust grains found in asteroids, comets, and moons. He uses UV light and electron sources to electrostatically charge dust grains, which he brings into contact with organic films, with the ultimate goal of understanding the potential toxic effects encountered by astronauts.
“It’s the fundamental science questions that keep us interested,” Schaible says. “What’s out there? How does it work? What can it tell us about our world, about ourselves? How can we extend our technology into space in a way that can make things better here at home?”
These are not rhetorical questions and Schaible expects to be part of the answers as he moves forward in his career, which is exactly the kind of thing that more experienced researchers like Thomas Orlando or Nick Hud want to hear: Going forward, the research is in good, eager, inquisitive hands.
“There’s something significant about this, about NASA funding great young researchers that want to become postdocs at Georgia Tech,” Hud says. “It’s the kind of support and investment that speaks well for the maturity of this discipline at Tech, about the depth and breadth of our research here.”
Video
Petit Institute NASA researchers
Future Moon Exploration (featuring Orlando lab)
Further Reading
NASA Comic Book (Astrobiology: The Story of our Search for Life in the Universe)
Astrobiology Rising at Georgia Tech
Unlocking the Mystery of Methane Clathrates
NASA Administrator Visits Georgia Tech
DNA Helix May have Arisen with Startling Ease
Coming Up

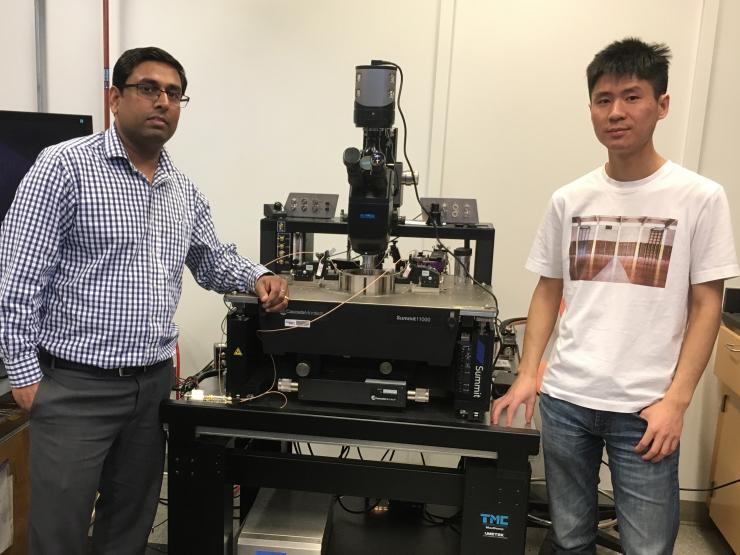
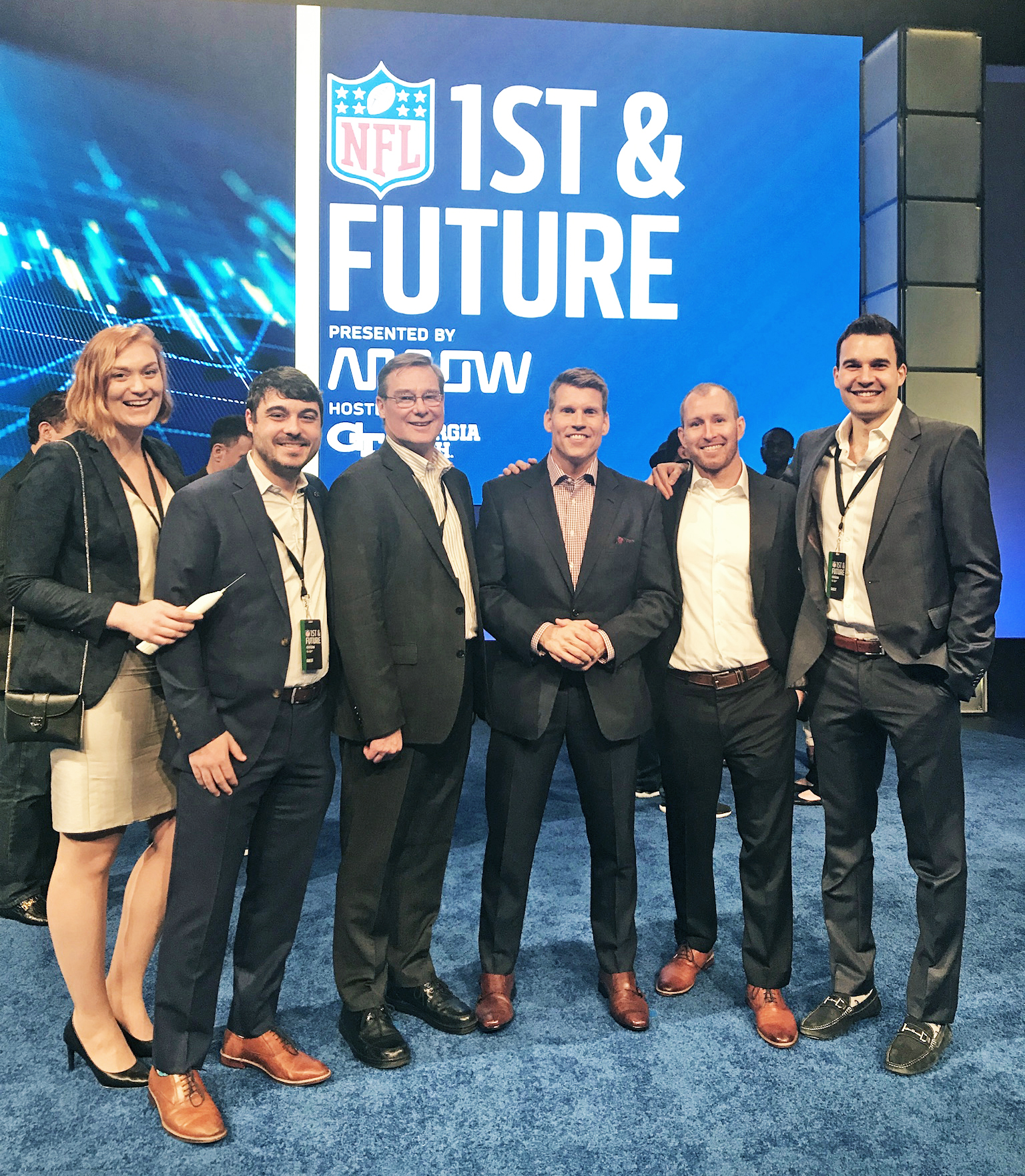
Jerry Grillo
Communications Officer II
Parker H. Petit Institute for
Bioengineering and Bioscience